Introduction
Marine pelagic fish constitute critically important resources globally. Lower-tropic level (LTL) pelagic species or “forage fish” account for over 30% of global fish landings (Smith et al., 2011). Lower-tropic level pelagic stocks (Atlantic herring [Clupea harengus (Linnaeus, 1758)], blue whiting [Micromesistius poutassou (Risso, 1827)], boarfish [Capros aper (Linnaeus, 1758)], Atlantic mackerel [Scomber scombrus (Linnaeus, 1758)] and Atlantic horse mackerel [Trachurus trachurus (Linnaeus, 1758)]) have always been an important component of the Irish fishing industry. While they dominate Irish landings in terms of tonnage, only Atlantic mackerel and Atlantic horse mackerel currently contribute considerably to the value of landings (Gerritsen & Lordan, 2014).
The level of demand for Atlantic herring, blue whiting and boarfish for direct human consumption does not match the volume in which they are landed. Therefore, these fish are commonly sold at low prices for reduction to fish meal and low-grade fish oil for animal feeds (Neiland, 2007; Alder et al., 2008; Egerton et al., 2017). However, researchers are investigating alternative processing methods, products and uses that could add value to these fish. Thorough and reliable knowledge of the compositional make-up of the raw material is imperative for processing and product development. Thus far, the published research in this area has principally focussed on single fraction compositional studies rather than providing complete, in-depth proximal composition data.
In the 1960s, at least 90% of all fish body oils were produced from different species of herrings (Lambertsen & Brækkan, 1965). Therefore, there is a knowledge base of lipid composition for this species. However, much of the work has focussed on Pacific (Huynh et al., 2007) or Baltic herring (Aro et al., 2000; Szlinder-Richert et al., 2010), or, alternatively, Atlantic herring fished off the North American coast (Ackman & Eaton, 1966; Ackman et al., 1967; Lane et al., 2011). There has been much less interest in the protein fraction of Atlantic herring, with only a few published studies investigating protein hydrolysates derived from this species (Sathivel et al., 2003; Beaulieu et al., 2009; Pampanin et al., 2012).
In contrast, research on processing and product development for blue whiting and boarfish has focussed on the protein fraction with little or no research on their lipid fraction (Huidobro & Tejada, 1995; Pérez-Mateos et al., 1997; Fernández-Martín et al., 1998; Cudennec et al., 2012; Nobile et al., 2016; Ojha et al., 2016; McLaughlin et al., 2017; Crowe et al., 2018; Egerton et al., 2018; Parthsarathy et al., 2019). Only two studies related to boarfish lipids could be found: a magazine article that briefly investigated the omega-3 content of boarfish (Gormley, 2015) and a peer-reviewed article that reported the lipid composition and fatty acids profile in selected fish meals, of which boarfish was an ingredient (Mika et al., 2016).
Thus far, there has been no published research on the mineral content of boarfish, whereas the mineral content of blue whiting and herring has been reported in a number of studies. Blue whiting was one of a selection of marine fish sampled in two studies that investigated the mineral content of fish from Norwegian and Mediterranean seas (Toppe et al., 2007). The Norwegian study also included Baltic herring. Other studies that have reported mineral content of herring have taken fish from off the coast of Alaska (Sathivel et al., 2003), Cameroon (Tenyang et al., 2014) and the Baltic Sea (Tahvonen et al., 2000; Marmon & Undeland, 2010). Location of fish development is a known factor that can influence mineral content (Martínez-Valverde et al., 2000). Despite this, no studies reporting the mineral content of these species fished in the Northeast Atlantic region could be found.
This study compares the proximate composition (protein, lipid, moisture and ash) of the LTL pelagic species blue whiting, boarfish and Atlantic herring (hereafter referred to as herring unless differentiation from Pacific herring is required) from Irish landings fished in the Northeast Atlantic region. It presents results of an in-depth analysis of the protein, lipid and mineral compositions, providing information that can be used to guide future processing and product development.
Materials and methods
Materials
Blue whiting, boarfish and herring samples were donated by Biomarine Ingredients Ireland Ltd (Monaghan, Ireland). The fish were caught off the north-west coast of Ireland in 2016 and immediately frozen at sea (−20°C). They were transported, frozen on ice, to the laboratory at Teagasc Food Research Centre, Moorepark, (Cork, Ireland) and were stored at −20°C until required. On thawing, whole fish were homogenised (Robot Coupe blixer 2, Robot-Coupe (UK) Ltd., Isleworth, England) individually to create a uniform mince of each fish. The mince was stored as aliquots (~25 g) at −20°C and defrosted as required prior to analysis.
Chemical reagents
Cyclohexane, hexane, heptane, methanol, propan-2-ol, formic acid, hydrochloric acid, 2 M hydrochloric acid in methanol and 25% sodium methoxide were purchased from Sigma Aldrich (Dublin, Ireland). Diethyl ether was purchased from Fisher Scientific (Dublin, Ireland). Triacylglycerol (TG) standard trinonadecanoin glyceride (C19:0) (part no. T-165), fatty acid methyl ester (FAME) standard methyl nonadecanoate (C19:0) (part no. N-19M) and FAME standard mix containing C10:0 to C24:1 methyl esters (part no. GLC 462) were purchased from Nu-Chek Prep Inc. (Waterville, MN, USA). Phospholipid (PL) standard 1,2-dinonadecanoyl-sn-glycero-3-phosphocholine (C19:0 PC) (part no. 850367P-AVL) was purchased from Stratech (Suffolk, England). Aminopropyl cartridges (500 mg, part no. 12102041) were obtained from Agilent Technologies Ireland Ltd. (Cork, Ireland).
Proximate composition
Proximate composition was determined for the whole individual fish (n = 30/fish species). Moisture content was determined gravimetrically after drying at 105°C for 24 h. Ash content was measured gravimetrically after incinerating the samples overnight in a furnace at 550°C. Total nitrogen content was determined by the macro-Kjeldahl method, and crude protein content was estimated by multiplying total nitrogen content by 6.25. Lipid content was determined gravimetrically after extraction following a modified Bligh and Dyer method (Smedes, 1999). Analyses of moisture and ash contents were performed in triplicate, while analyses of protein and fat contents were performed in duplicate.
Amino acid composition
An aliquot (10 g) of whole homogenised fish (n = 6) of each species was pooled before being hydrolysed in 6 N hydrochloric acid at 110°C for 23 h and total amino acid content was determined for the resulting hydrolysates according to McDermott et al. (2016). Amino acids were quantified using a Jeol JLC-500/V amino acid analyser (Jeol (UK) Ltd., Welwyn Garden City, England) fitted with a Jeol Na+ high-performance cation-exchange column.
Comparative analyses were carried out between the amino acid composition of the three fish species, the dietary essential amino acid (EAA) recommendations for human adults (FAO/WHO/UNU, 2007) and Atlantic salmon feeds (NRC, 2011) and, the amino acid composition of a range of common food protein sources: milk, whey protein hydrolysate (WPH), egg, soy and pea, as outlined in previously published research (Gilbert et al., 2011; Medhammar et al., 2012; Day, 2013; Morato et al., 2013).
Lipid composition
Total lipids were extracted from whole homogenised fish samples (n = 3/fish species) following a modified Bligh and Dyer method (Smedes, 1999). Lipid classes (TG, free fatty acids [FFA], PL and glycolipids [GL]) were separated by solid-phase extraction following the methods described in O’Callaghan et al. (2016) with some modifications. Briefly, 500 mg aminopropyl cartridges were preconditioned with 10 mL of heptane. The lipid extract was added to the column, a vacuum was applied and the TG were eluted. The remaining TG were removed using 10 mL of 20% diethyl ether/hexane (v/v). Next, FFA were eluted using 5 mL of 2% formic acid/diethyl ether (v/v) followed by GL and PL using 5 mL of acetone/isopropanol (1:1, v/v) and 5 mL of methanol, respectively. The isolated lipid classes were dried down under a flow of nitrogen and percent proportion of total lipids was calculated gravimetrically. Subsequently, the entire PL extract was redissolved in hexane (500 μL) and an aliquot (200 mg) of TG was redissolved in hexane (25 mL).
The methyl esters and standard curve for FAME analysis, along with in-run quality control samples, were prepared using an Agilent 7696A Sample Prep Workbench (Agilent Technologies Ireland Ltd). TG and PL extracts were transmethylated by mixing with 0.5 M sodium methoxide in anhydrous methanol (24:1, v/v) at 25°C (Carvalho & Malcata, 2005). The reaction was neutralised with 0.5 M hydrochloric acid in methanol and transmethyl esters in the top layer were transferred to amber glass vials for gas chromatography (GC) analysis. Each sample included the internal standard nonadecanoic acid (C19:0). FAME were analysed on an Agilent 7890B GC, equipped with a flame ionisation detector (FID), GC80 autosampler and a multimode inlet injector (Agilent Technologies Ireland Ltd.). The column was a Chrompack CP Sil 88 column (100 m × 0.25 mm internal diameter, 0.25 mm phase thickness; JVA Analytical, Dublin, Ireland), and helium was used as the carrier gas, which was held at a constant flow of 1.2 mL/min. The injector was held at 300°C for the entire run and was operated in the split/splitless mode using a split of 1:20. The inlet liner used was a wool-packed liner (part no. 392611953; Agilent Technologies Ireland Ltd.). The oven cycle was programmed initially at 80°C for 8 min followed by an increase of 8.5°C/min to a final column temperature of 200°C. The total run time was 72 min. The FID was operated at 300°C. Peak integration was performed using the Agilent OpenLAB Chemstation software (Version A.01.06.111, Cork, Ireland).
Identification of individual FAME was achieved by comparison of retention times with pure FAME standards and quantification against the internal standards. Results are expressed as g/100 g of total FAME.
Comparative analyses were carried out between the lipid composition of the TG fraction of the three fish species and the composition of the total lipids of important marine fish in the Mediterranean Sea, Aegean Sea, Black Sea and the Pacific Ocean, as reported in three published research articles (Özogul & Özogul, 2007; Huynh & Kitts, 2009; Prato & Biandolino, 2012). Specific consideration was given to the sums of poly-unsaturated fatty acids (PUFA) and omega-3 fatty acids and relative concentrations of docosahexaenoic acid (DHA; C22:6n3) and eicosapentaenoic acid (EPA; C20:5n3).
Mineral composition
An aliquot (20 g) of whole homogenised fish (n = 6) of each species was pooled and sent to an external laboratory (ALS Life Sciences, Laois, Ireland) for mineral and heavy metal analysis. Briefly, all samples were homogenised and mineralised by acids and hydrogen peroxide on arrival at the external laboratory and subsequently analysed using the following methods. Calcium, magnesium, phosphorus, potassium, sodium, iron and zinc were determined by atomic emission spectrometry with inductively coupled plasma and stoichiometric calculations of concentrations of compounds from measured values (CZ_SOP_D06_02_001 [US EPA 200.7, CSN EN ISO 11885, samples prepared as per CZ_SOP_D06_02_J02 chap. 10.17.1, 10.17.2, 10.17.4, 10.17.7, 10.17.8]). Arsenic, cadmium, copper, lead and selenium were similarly determined using mass spectrometry (CZ_SOP_D06_02_002 [US EPA 200.8, CSN EN ISO 17294-2, CSN EN 15111, samples prepared as per CZ_SOP_D06_02_J02 chap. 10.17.1, 10.17.2, 10.17.4, 10.17.7, 10.17.8]). Mercury was determined by atomic absorption spectrometry (CZ_SOP_D06_02_003 [CSN 46 5735, CSN 75 7440, CL, PhEur, USP samples prepared as per CZ_SOP_D06_02_J02 chap.10.1 to 10.17.14, 10.20].
Comparative analyses were carried out between the mineral composition of the three fish species and the recommended daily intake for adults, as outlined by the United Nations’ World Health Organization (UN’s WHO) and the Institute of Medicine (Institute of Medicine, 1997; FAO/WHO, 1998; WHO, 2012a, 2012b). The total heavy metals found in the three fish species were also compared with the maximum allowable limit (MAL) in fish for human consumption as specified by various regulatory bodies (Nauen, 1983; FAO/WHO, 1998; Bosch et al., 2016).
Statistical analysis
Statistical analyses were carried out using GraphPad Prism Version 7.02 (San Diego, CA, USA). Proximate composition data (n = 30) were submitted to analysis of variance (ANOVA) and pairwise comparisons were conducted by Tukey’s test. Pearson’s correlation was used to investigate the relationship between total moisture and lipids of the fish. Lipid composition data (n = 3) were submitted to ANOVA and pairwise comparisons were conducted by Tukey’s test. Significance level was determined at the 95% probability level.
Results
Proximate composition
Significant differences in the percentage of whole-body weight were found in moisture, ash, protein and lipid contents of the three species (Figure 1). Of the four fractions, the least differences were recorded in the protein content of the three fish species. However, the proportion of protein content was lowest in boarfish, which was found to be significantly lower than the proportion of protein in blue whiting (P < 0.05) but not in herring.
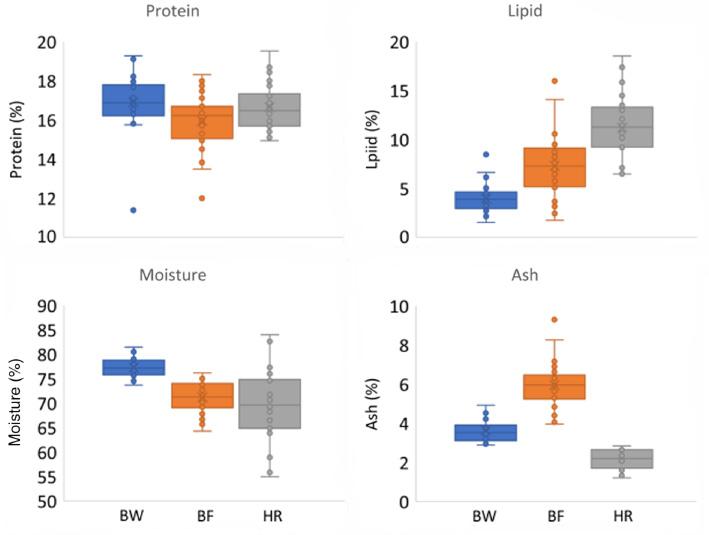
The proximate composition (protein, lipid, moisture and ash) of blue whiting (BW), boarfish (BF) and herring (HR) as percentage of whole-body wet weight (n = 30).
Herring had a significantly higher lipid content compared with boarfish (P < 0.001), which in turn had a significantly higher lipid content than blue whiting (P < 0.001; Figure 1). Comparing the total lipid content of these three species with that of other marine fish reported in the literature, Atlantic herring had the highest total lipid content while boarfish and blue whiting were fattier than 89% and 69% of fish, respectively (Table 1).
Total lipid content (% wet weight) of important marine fish in the Mediterranean Sea, Aegean Sea, Black Sea and the Pacific Ocean as reported in three published research articles as well as the results from this study for blue whiting, Atlantic herring and boarfish (in bold).
Fish species | % Wet weight |
---|---|
Pacific hake | 0.73 ± 0.291 |
Common sole | 0.74 ± 0.052 |
Walleye pollock | 0.79 ± 0.171 |
Red scorpion fish | 0.87 ± 0.042 |
Turbot | 1.30 ± 0.122 |
Scad | 1.37 ± 0.252 |
Pandora | 1.67± 0.022 |
Salema | 1.67 ± 0.113 |
Black goby | 1.92 ± 0.053 |
Mullet | 2.09 ± 0.072 |
Grass goby | 2.13 ± 0.133 |
Seabass | 2.33 ± 0.103 |
Picarel | 2.84 ± 0.203 |
Sand smelt | 2.94 ± 0.163 |
Surf smelt | 3.10 ± 1.121 |
Canary rock fish | 3.31 ± 0.371 |
Sardine | 3.47 ± 0.252 |
Two-banded seabream | 3.61 ± 0.093 |
Bogue | 3.64 ± 0.222 |
Blue whiting | 3.94 ± 1.40 |
Pink salmon | 3.95 ± 0.791 |
Grey wrasse | 4.15 ± 0.103 |
Capelin | 5.10 ± 0.891 |
Bogue | 5.62 ± 0.113 |
Golden grey mullet | 5.96 ± 0.133 |
Sardine | 6.43 ± 1.641 |
Boarfish | 7.28 ± 3.22 |
Red mullet | 8.12 ± 0.273 |
Pacific herring | 10.78 ± 0.681 |
Atlantic herring | 11.20 ± 2.96 |
Moisture content was highest in blue whiting and lowest in herring. Herring also showed the greatest variation in moisture content between individuals (range: 55.0–84.0%). A negative correlation between moisture content and lipid content of individuals in all three species was clearly observed (r = −0.5776, −0.8138 and −0.8065 for blue whiting, boarfish and herring, respectively, P < 0.001; Figure 2).
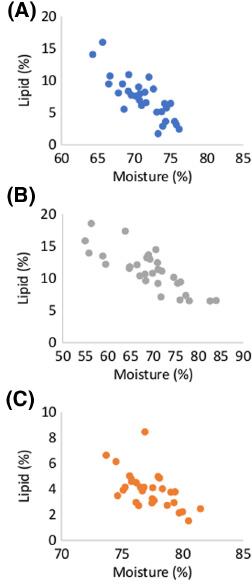
The correlation in whole-body moisture (%) and lipid (%) contents of (a) blue whiting, (b) boarfish and (c) herring (n = 30).
Finally, boarfish was found to have the highest ash content. This was significantly higher than the ash content of blue whiting (P < 0.001) which in turn was significantly higher than that of herring (P < 0.001).
Amino acid composition
The amino acid profiles of the three fish species and the composition of a range of common food protein sources as reported in previous publications are outlined in Table 2. The amino acid profiles of the three fish were similar. However, for most EAA, blue whiting had the lowest concentrations. Herring had the highest concentration of the branched-chain amino acids (BCAA): leucine, isoleucine and valine. These levels of BCAA were similar to those found in egg, soy and pea. However, milk and WPH had higher levels of BCAA. Of the three fish species, boarfish had the optimum EAA ratio for human and salmon requirements. Cysteine was a significantly limiting amino acid of these fish species in terms of human nutrition. However, they all contained high concentrations of methionine, a precursor of cysteine.
Recommended essential amino acid (EAA) daily intake per kilogramme weight of an adult and recommended EAA composition ratio (mg/g protein) for human adults and Atlantic salmon feed compared with the EAA composition (mg/g protein) found in whole blue whiting (BW), boarfish (BF) and herring (HR) (pooled samples, n = 6) as well as a range of common food protein sources: milk, whey protein hydrolysate (WPH), egg, soy and pea.
| Amino acid requirements | BW | BF | HR | Milk4 | WPH5 | Egg6 | Soy7 | Pea7 | ||
---|---|---|---|---|---|---|---|---|---|---|---|
| Humans (adults)1
| Salmon2,3
|
| ||||||||
Amino acid | mg/kg per day | mg/g protein | mg/g feed | mg/g protein | |||||||
Arginine | – | – | 17 | 57 | 68 | 62 | 23 | 23 | 81 | 73 | 102 |
Histidine | 10 | 15 | 7 | 27 | 24 | 44 | 23 | 13 | 25 | 26 | 25 |
Isoleucine | 20 | 30 | 12 | 32 | 39 | 44 | 50 | 70 | 53 | 46 | 46 |
Leucine | 39 | 59 | 21 | 57 | 69 | 80 | 81 | 102 | 86 | 79 | 73 |
Lysine | 30 | 45 | 24 | 62 | 77 | 85 | 43 | 95 | 73 | 65 | 81 |
Methionine | 10 | 16 | – | 35 | 40 | 44 | 23 | 25 | 30 | 13 | 10 |
Cysteine | 4 | 6 | – | 9 | 2 | 2 | 5 | 16 | 22 | 13 | 12 |
Methionine + cysteine | 15 | 22 | 12 | 44 | 42 | 46 | 28 | 41 | 52 | 26 | 22 |
Phenylalanine + Tyrosine | 25 | 30 | 25 | 51 | 63 | 48 | 91 | – | 94 | 82 | 78 |
Threonine | 15 | 23 | 15 | 35 | 41 | 47 | 44 | 74 | 44 | 39 | 44 |
Tryptophan | 4 | 6 | 3 | – | – | – | 23 | – | 13 | 13 | 10 |
Valine | 26 | 39 | 17 | 42 | 46 | 56 | 59 | 58 | 68 | 49 | 51 |
Lipid composition
The average total lipid contents of the fish used for lipid composition analysis were 4.7 ± 1.1%, 6.1 ± 1.0% and 10.8 ± 0.8% for blue whiting, boarfish and herring, respectively. The total lipids were principally composed of TG (Figure 3). Herring had the highest amount of TG and this difference was found to be significant (P < 0.001) compared with blue whiting and boarfish. Levels of PL and GL detected in the oil were similar for all three fish species. FFA made up a similar proportion of total lipids in blue whiting and boarfish, whereas in herring they were detected at a significantly lower concentration (P < 0.001; Figure 3).
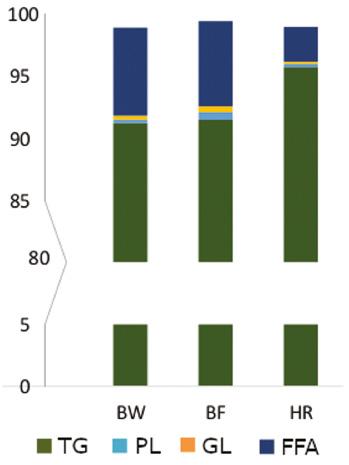
The proportion of four lipid classes (triacylglycerols [TG], phospholipids [PL], glycolipids [GL] and free fatty acids [FFA]) obtained from the total lipids of blue whiting (BW), boarfish (BF) and herring (HR) (n = 3).
The fatty acid compositions of the TG and PL fractions were investigated using a GC-FID to analyse the derivatised FAME. The results of the TG fraction are outlined in Table 3. Palmitic acid (C16:0) was the fatty acid found in the greatest concentration in all three fish species. This was followed by oleic acid (C18:1n9) and DHA. The concentration of only one of the TG fatty acids varied significantly between the three fish: myristic acid (C14:0) was found at a similar concentration in boarfish and herring while blue whiting had a significantly lower concentration (P < 0.05; Table 3).
Triacylglycerol fatty acid composition (corrected FID response area relative percentages) and the sums of triacylglycerols, grouped by the level of saturation and double bond location, of blue whiting, boarfish and herring (n = 3). Values with different superscripts in the same row are significantly different.
Blue whiting | Boarfish | Herring | Significance (P<) | |
---|---|---|---|---|
SFA | ||||
C10:0 | 0.01 ± 0.0 | 0.01 ± 0.00 | 0.01 ± 0.01 | ns |
C12:0 | 0.07 ± 0.01 | 0.09 ± 0.02 | 0.12 ± 0.04 | ns |
C14:0 | 6.31 ± 0.61a | 10.56 ± 4.55b | 9.65 ± 1.90b | 0.01 |
C16:0 | 21.89 ± 1.06 | 20.15 ± 2.49 | 23.58 ± 1.91 | ns |
C18:0 | 2.76 ± 0.36 | 4.44 ± 0.93 | 3.95 ± 0.32 | ns |
C20:0 | 0.15 ± 0.04 | 0.28 ± 0.11 | 0.27 ± 0.10 | ns |
C22:0 | 0.07 ± 0.01 | 0.08 ± 0.02 | 0.08 ± 0.02 | ns |
C24:0 | – | 0.05 ± 0.00 | 0.05 ± 0.00 | ns |
MUFA | ||||
C12:1 | 0.02 ± 0.00 | – | – | ns |
C14:1 | 0.18 ± 0.02 | 0.13 ± 0.04 | 0.13 ± 0.02 | ns |
C16:1 | 7.26 ± 0.58 | 4.80 ± 2.94 | 6.78 ± 1.37 | ns |
C18:1n9 | 20.79 ± 2.87 | 19.45 ± 5.24 | 20.97 ± 4.88 | ns |
C18:1n11 | 4.87 ± 0.95 | 2.37 ± 0.22 | 2.27 ± 0.24 | ns |
C20:1 | 9.24 ± 1.65 | 9.82 ± 4.88 | 7.77 ± 4.76 | ns |
C22:1n9 | 0.78 ± 0.66 | 1.39 ± 0.88 | 0.85 ± 0.35 | ns |
C24:1 | 0.05 ± 0.01 | 0.11 ± 0.06 | 0.08 ± 0.02 | ns |
PUFA | ||||
C18:2n6 | 1.51 ± 0.07 | 1.54 ± 0.19 | 1.39 ± 0.11 | ns |
C18:3n6 | 0.16 ± 0.01 | 0.11 ± 0.01 | 0.12 ± 0.02 | ns |
C18:3n3 | 0.91 ± 0.22 | 0.86 ± 0.18 | 0.72 ± 0.08 | ns |
C20:2 | 0.32 ± 0.10 | 0.37 ± 0.24 | 0.32 ± 0.13 | ns |
C20:3n6 | 0.06 ± 0.01 | 0.12 ± 0.02 | 0.11 ± 0.02 | ns |
C20:4n6/C20:3n3 | 1.71 ± 0.20 | 1.87 ± 0.28 | 1.48 ± 0.21 | ns |
C20:5n3 | 8.07 ± 1.31 | 6.37 ± 1.74 | 5.85 ± 1.24 | ns |
C22:2 | 0.06 ± 0.04 | 0.07 ± 0.01 | 0.03 ± 0.02 | ns |
C22:4 | 0.19 ± 0.03 | 0.72 ± 0.51 | 0.47 ± 0.31 | ns |
C22:5n3 | 0.76 ± 0.23 | 1.61 ± 0.77 | 1.19 ± 0.48 | ns |
C22:6n3 | 11.88 ± 3.43 | 13.30 ± 0.49 | 11.82 ± 1.59 | ns |
SFA ∑ | 31.3 ± 0.9a | 35.6 ± 3.1b | 37.7 ± 0.4b | 0.001 |
MUFA ∑ | 43.2 ± 1.5a | 38.1 ± 2.0b | 38.9 ± 1.5b | 0.001 |
PUFA ∑ | 25.6 ± 2.3ab | 27.0 ± 1.2a | 23.5 ± 1.1b | 0.05 |
PUFA/SFA | 0.8 ± 0.1 | 0.8 ± 0.1 | 0.6 ± 0.0 | ns |
Omega 3 ∑ | 21.6 ± 2.2 | 22.1 ± 1.3 | 19.6 ± 0.9 | ns |
Omega 6 ∑ | 2.3 ± 0.1 | 2.9 ± 0.7 | 2.4 ± 0.4 | ns |
Omega 3:omega 6 | 9.4 ± 1.2 | 8.2 ± 2.6 | 8.2 ± 1.1 | ns |
DHA:EPA | 1.6 ± 0.6 | 2.3 ± 0.7 | 2.2 ± 0.8 | ns |
DHA = docosahexaenoic acid; EPA = eicosapentaenoic acid; FID = flame ionisation detector; MUFA = mono-unsaturated fatty acids; ns = not significant; PUFA = poly-unsaturated fatty acids; SFA = saturated fatty acids.
Of the fatty acids analysed, total saturated fatty acids (SFAs) made up over one-third of total fatty acids detected in boarfish and herring. SFAs in blue whiting were at a significantly lower concentration (P < 0.001). Mono-unsaturated fatty acids (MUFA) were highest in blue whiting, significantly more so than boarfish or herring (P < 0.01). PUFA composed approximately one-quarter of total fatty acids in all three fish species, but boarfish had a significantly higher relative abundance compared with herring (P < 0.05; Table 3). No significant differences in the relative abundances of DHA or EPA, or the sums of omega-3s and omega-6s, were found between the three fish species in the TG fraction.
It can be seen in Table 4 that blue whiting, boarfish and Atlantic herring had low relative abundance of PUFA and omega-3 fatty acids compared with a range of other important marine fish. However, DHA and EPA relative abundance levels in Atlantic herring were higher compared with 20% of the other fish, while boarfish had higher relative abundance levels of DHA and EPA compared with one-third of the fish. Interestingly, blue whiting had higher DHA levels compared with 25% of the fish but was in the top third of fish when comparing EPA concentrations (Table 4).
The sums of PUFA and omega-3 fatty acids and relative concentrations of DHA and EPA from total lipids (%) of important marine fish in the Mediterranean, Aegean and Black Seas and the Pacific Ocean as reported in three published research articles and, the results from this study for blue whiting, Atlantic herring and boarfish (triacylglycerols fraction only, indicated in bold).
PUFA ∑ | Omega-3 ∑ | DHA | EPA | |
---|---|---|---|---|
Herring | 23.5 | 19.6 | 11.8 | 5.9 |
Pacific herring1 | 24.2 | 20.6 | 8.6 | 8.7 |
Capelin1 | 24.6 | 20.9 | 10.0 | 7.9 |
Mullet2 | 24.8 | 21.7 | 7.7 | 10.5 |
Blue whiting | 25.6 | 21.6 | 11.9 | 8.1 |
Boarfish | 27.0 | 22.1 | 13.3 | 6.4 |
Bogue2 | 27.5 | 26.0 | 18.7 | 5.1 |
Red mullet3 | 27.7 | 20.8 | 12.1 | 7.2 |
Grey wrasse3 | 28.8 | 21.1 | 9.9 | 6.8 |
Golden grey mullet3 | 28.9 | 21.5 | 10.8 | 6.1 |
Sardine1 | 31.0 | 27.6 | 13.3 | 11.7 |
Grass goby3 | 31.8 | 22.9 | 12.3 | 5.0 |
Pandora2 | 32.0 | 30.3 | 21.9 | 5.3 |
Picarel3 | 32.1 | 25.2 | 14.7 | 7.2 |
Salema3 | 32.1 | 26.8 | 17.4 | 7.2 |
Seabass3 | 32.5 | 23.1 | 13.8 | 6.9 |
Common sole2 | 33.6 | 31.1 | 18.7 | 7.7 |
C.T. seabream3 | 34.0 | 27.0 | 17.2 | 7.5 |
Black goby3 | 34.2 | 23.4 | 12.5 | 6.1 |
Sand smelt3 | 34.7 | 26.1 | 15.7 | 8.6 |
Canary rock fish1 | 37.1 | 33.8 | 18.2 | 11.6 |
Pink salmon1 | 38.9 | 35.0 | 19.3 | 8.2 |
Turbot2 | 41.1 | 38.4 | 30.3 | 5.3 |
Red scorpion fish2 | 41.6 | 37.0 | 28.0 | 4.7 |
Pacific hake1 | 43.5 | 38.4 | 22.1 | 12.9 |
Surf smelt1 | 44.5 | 38.8 | 20.7 | 12.9 |
Scad2 | 46.4 | 43.7 | 36.2 | 5.4 |
Walleye pollock1 | 47.6 | 44.9 | 29.0 | 12.4 |
Sardine2 | 56.8 | 51.2 | 32.7 | 11.4 |
DHA = docosahexaenoic acid; EPA = eicosapentaenoic acid; PUFA = poly-unsaturated fatty acids.
In contrast to the TG fatty acid composition, the PL fatty acid composition of the three fish had several significant variations (Table 5). Palmitic acid, DHA and oleic acid were also the three most abundant fatty acids detected in the PL fraction. Although DHA was the most abundant fatty acid in all three fish, it was detected at a significantly higher relative concentration in herring compared with blue whiting and boarfish (P < 0.001). EPA was detected in herring and blue whiting at similar concentrations, whereas boarfish only contained approximately half of this, significantly less than the other two fish species (P < 0.001). Significant differences in the relative concentration of palmitic acid, stearic acid (C18:0), oleic acid and α-linolenic acid (ALA) (C18:3n3) were also found between the three fish (Table 5).
Phospholipid fatty acid composition (corrected FID response area relative percentages) and the sums of phospholipid fatty acids, grouped by the level of saturation and double bond location, detected in blue whiting, boarfish and herring (n = 3). Values with different superscripts in the same row are significantly different.
Blue whiting | Boarfish | Herring | Significance (P<) | |
---|---|---|---|---|
SFA | ||||
C10:0 | 0.01 ± 0.00 | 0.01 ± 0.00 | 0.01 ± 0.01 | ns |
C12:0 | 0.25 ± 0.31 | 0.01 ± 0.00 | 0.02 ± 0.01 | ns |
C14:0 | 2.07 ± 0.55 | 2.07 ± 0.21 | 1.50 ± 0.39 | ns |
C16:0 | 24.86 ± 3.31a | 26.79 ± 1.12a | 31.18 ± 2.49b | 0.001 |
C18:0 | 2.98 ± 0.67a | 7.13 ± 1.16b | 2.40 ± 0.08a | 0.001 |
C20:0 | 0.06 ± 0.01 | 0.16 ± 0.05 | 0.07 ± 0.01 | ns |
C22:0 | 0.07 ± 0.01 | 0.11 ± 0.01 | 0.04 ± 0.01 | ns |
C24:0 | 0.05 ± 0.00 | 0.15 ± 0.00 | – | ns |
MUFA | ||||
C12:1 | – | – | – | ns |
C14:1 | 0.01 ± 0.00 | 0.02 ± 0.01 | 0.01 ± 0.01 | ns |
C16:1 | 2.28 ± 0.40 | 2.09 ± 0.09 | 2.18 ± 0.37 | ns |
C18:1n9 | 19.33 ± 1.55a | 13.96 ± 0.58b | 9.50 ± 1.31c | 0.001 |
C18:1n11 | 3.08 ± 0.36 | 1.74 ± 0.10 | 2.29 ± 0.28 | ns |
C20:1 | 2.26 ± 0.31 | 2.14 ± 0.56 | 0.75 ± 0.22 | ns |
C22:1n9 | 0.28 ± 0.04 | 0.45 ± 0.24 | 0.09 ± 0.01 | ns |
C24:1 | 0.05 ± 0.00 | 0.19 ± 0.05 | 0.04 ± 0.01 | ns |
PUFA | ||||
C18:2n6 | 0.99 ± 0.08 | 0.87 ± 0.18 | 0.53 ± 0.09 | ns |
C18:3n6 | 0.10 ± 0.02 | 0.09 ± 0.03 | 0.07 ± 0.02 | ns |
C18:3n3 | 0.36 ± 0.05a | 0.17 ± 0.02b | 0.20 ± 0.05b | 0.05 |
C20:2 | 0.20 ± 0.03 | 0.24 ± 0.04 | 0.10 ± 0.01 | ns |
C20:3n6 | 0.11 ± 0.04 | 0.09 ± 0.02 | 0.08 ± 0.01 | ns |
C20:4n6/C20:3n3 | 0.28 ± 0.04 | 0.45 ± 0.24 | 0.09 ± 0.01 | ns |
C20:5n3 | 11.96 ± 0.64a | 5.76 ± 1.15b | 10.26 ± 0.74a | 0.001 |
C22:2 | 0.06 ± 0.02 | 0.04 ± 0.00 | – | ns |
C22:4 | 0.24 ± 0.04 | 1.03 ± 0.21 | 0.37 ± 0.12 | ns |
C22:5n3 | 1.08 ± 0.34 | 1.63 ± 0.18 | 0.49 ± 0.03 | ns |
C22:6n3 | 24.63 ± 2.19a | 29.35 ± 2.24ab | 35.38 ± 4.75b | 0.001 |
SFA ∑ | 30.3 ± 3.1a | 36.3 ± 1.1b | 35.2 ± 2.9b | 0.05 |
MUFA ∑ | 27.3 ± 1.6a | 20.6 ± 0.7b | 14.9 ± 1.8c | 0.001 |
PUFA ∑ | 42.5 ± 2.5a | 43.1 ± 1.6ab | 49.9 ± 4.3b | 0.05 |
PUFA/SFA | 1.4 ± 0.2 | 1.2 ± 0.1 | 1.4 ± 0.2 | ns |
Omega-3 ∑ | 38.0 ± 2.4a | 36.9 ± 1.1a | 46.3 ± 4.4b | 0.001 |
Omega-6 ∑ | 1.7 ± 0.2 | 2.4 ± 0.1 | 1.1 ± 0.2 | ns |
Omega-3:omega-6 | 22.7 ± 2.5a | 15.7 ± 1.1a | 43.0 ± 10.9b | 0.001 |
DHA:EPA | 2.1 ± 0.2 | 5.4 ± 1.3 | 3.5 ± 0.7 | ns |
DHA = docosahexaenoic acid; EPA = eicosapentaenoic acid; FID = flame ionisation detector; MUFA = mono-unsaturated fatty acids; ns = not significant; PUFA = poly-unsaturated fatty acids; SFA = saturated fatty acids.
Phospholipid levels of SFA were similar to the TG fraction. However, the relative abundances of the total MUFA and PUFA were contrasting; MUFA comprised a lower fraction of total PL, whereas PUFA made up the largest fraction. Herring contained the highest relative concentration of PUFA. The relative concentration of PUFA in herring was significantly higher than that of blue whiting but not that of boarfish (P < 0.05). The difference in PUFA relative concentration is the result of higher relative levels of omega-3 fatty acids in herring (Table 5).
Mineral composition
The mineral and heavy metal contents in the three fish species are outlined in Tables 6 and 7, respectively. Calcium was found at the greatest concentration in blue whiting and boarfish, while herring only contained approximately one-tenth of what was detected in the other fish species. Phosphorus was the second most prevalent mineral and followed a similar trend to calcium, in terms of the relative concentration levels in the different fish species, with herring containing approximately half the level of the other two fish species. The calcium and phosphorus contents in 100 g of blue whiting and boarfish were above the recommended daily intake for adults (Table 6). Sodium content of all fish was similar and within the recommended daily intake for adults. All other minerals analysed were detected at levels significantly below the recommended daily intake for adults. Particularly, selenium was detected at very low levels.
The mineral contents of whole blue whiting, boarfish and herring (mg/100 g) and the recommended daily intake for adults (mg/d)
mg/100 g | mg/d | ||||
---|---|---|---|---|---|
| |||||
Blue whiting | Boarfish | Herring | Male (19–65 yr) | Female (19–50 yr) | |
Calcium | 1,090.00 | 1,520.00 | 272.00 | 1,0001 | 1,0001 |
Iron | 1.07 | 2.83 | 2.06 | 9–271 | 20–591 |
Magnesium | 47.50 | 62.10 | 40.70 | 2601 | 2201 |
Phosphorus | 622.00 | 789.00 | 363.00 | 7002 | 7002 |
Potassium | 267.00 | 112.00 | 252.00 | 3,5103 | 3,5103 |
Selenium | 0.06 | 0.05 | 0.04 | 341 | 261 |
Sodium | 257.00 | 282.00 | 218.00 | 200–5004 | 200–5004 |
Zinc | 1.29 | 5.57 | 1.33 | 4–141 | 3–101 |
The heavy metal contents (mg/kg) of whole blue whiting, boarfish and herring and the maximum allowable limit as specified by various regulatory bodies
mg/kg | Blue whiting | Boarfish | Herring | MAL |
---|---|---|---|---|
Arsenic | 2.44 | 1.34 | 1.62 | 3.001 |
Cadmium | 0.07 | 0.124 | <0.04 | 1.001 |
Copper | 0.71 | 0.41 | 0.61 | 30.002 |
Lead | <0.05 | <0.05 | <0.05 | 0.203 |
Mercury | 0.073 | 0.068 | 0.047 | 0.501 |
Zinc | 12.90 | 55.70 | 13.30 | 30.002 |
All heavy metals detected were below the MAL specified by various regulatory bodies, except for the zinc levels found in boarfish. Zinc in boarfish was at 55.70 mg/kg and the MAL for zinc is at 30 mg/kg for fish (Nauen, 1983). Boarfish also had the highest level of cadmium. Arsenic, copper and mercury were at similar levels in all fish, although they were highest in blue whiting (Table 7).
Discussion
This study aimed to provide a detailed report of the proximate composition of three LTL pelagic fish species: blue whiting, boarfish and herring. It focussed on these species caught in the Northeast Atlantic region, for which there are no such published data to-date.
These fish species were found to be high in protein. The protein was a significant fraction of whole-body raw material when comparing it with other common protein sources. Whole milk contains approximately 3.2% protein, of which whey protein comprises only 20% (Medhammar et al., 2012). Eggs contain approximately 12% protein, while common plant protein sources such as soy and pea may contain 20–40% protein (Day, 2013; Marsh et al., 2013). When considering the efficacy of a raw material for protein powder production, total protein content and the amino acid profile should be considered. There are 20 standard proteinogenic amino acids, of which nine are EAA and need to be acquired in the diet. Protein synthesis is stimulated by EAA alone (Helms et al., 2014) and for dietary protein to be well incorporated into new protein it is necessary for the EAA to be at a ratio that resembles that of the protein to be synthesised, for example, human or animal muscle (Gilbert et al., 2011). Furthermore, within the EAA, the BCAA (valine, leucine and isoleucine), and particularly leucine, stimulate protein synthesis (Kimball & Jefferson, 2006). Egg was previously considered an excellent source for fulfilling EAA requirements and was traditionally used as the standard of comparison for measuring protein quality (Layman & Rodriguez, 2009). Likewise, WPH has become a popular raw material for protein powders marketed towards sports nutrition because of its high levels of BCAA, especially leucine. These foods are excellent sources of protein and BCAA. The amino acid ratio of WPH, however, is not balanced to the requirements of human nutrition. All EAA are required together in adequate quantities and proper proportions for protein synthesis. A deficit in any one EAA limits protein synthesis (Srikantia, 1981). Thus, the excess proportions of leucine in WPH cannot be fully utilised unless mixed with other proteins that provide higher levels of the limiting EAA in WPH.
Proteins from both pea and boarfish have the closest matching EAA ratio to salmon requirements. However, salmon are naturally carnivorous and plant proteins can contain antinutritional factors that have been shown to negatively affect salmon health and growth (Francis et al., 2001; Bendiksen et al., 2011; Dalsgaard et al., 2012). Therefore, of the foods compared here, boarfish protein would be considered the optimum protein source for use in salmon aquafeeds. In terms of human nutrition, of the foods compared here, milk had the closest matching EAA ratio to adult human requirements, followed closely by egg. Boarfish and blue whiting also had EAA ratios that were close to adult human requirements and, interestingly, there have been some studies reporting that fish proteins demonstrate higher satiating effects than other protein sources (Uhe et al., 1992; Borzoei et al., 2006; Pal & Ellis, 2010). Thus far, intervention studies comparing the effects of different protein sources on body weight have proved inconclusive (Gilbert et al., 2011). However, this is an area that is becoming increasingly important, especially in Western society and further investigation using standardised techniques would benefit this area of research.
Considering the total protein content and the amino acid profiles of blue whiting, boarfish and herring, these LTL fish would be ideal raw material for protein powder production destined for human consumption or the aquaculture industry. To optimise a product destined for human nutrition, protein derived from herring, and to a lesser extent blue whiting and boarfish, would benefit from mixing with other proteins (such as WPH) to create a product with an EAA ratio that more closely matches that recommended for humans.
Around 1 million tonnes of fish oil are produced every year (Ytrestøyl et al., 2011). Fish oils are generally considered to contain high levels of PUFA. PUFA have been associated with several different health benefits, the principal ones being improved cardiovascular health as well as healthy brain and eye functions. The active metabolites in fish oils are primarily the omega-3 PUFA, EPA and DHA, which are synthesised de novo in humans from the precursor essential fatty acid ALA (18:3n-3; Shahidi & Ambigaipalan, 2018). However, it is an inefficient pathway, and EPA and DHA are, therefore, generally considered as essential nutrients (Robertson et al., 2013). Marine fish build up high levels of PUFA, especially EPA and DHA, via their food chains which begin with PUFA-synthesising algae (Lenihan-Geels et al., 2013). Fish oils are hence valuable nutrients, highly sought after as supplements for humans and animals.
Fish are classified as “fatty fish” if their lipid content is above 8% of their body weight and moderately fatty if it is between 4 and 8% (Tanakol et al., 1999). Therefore, from the results of this study, herring is considered a fatty fish, whereas blue whiting and boarfish are considered to be moderately fatty fish. Herring was the species with the highest levels of total lipids, the highest proportion of TG and the lowest level of FFA. Free fatty acids are less stable than other oil fractions and are therefore more prone to oxidation and to turning rancid (Chatterjee & Judeh, 2016). Thus, the proportion of FFA is a key feature linked with the quality and commercial value of oils and fats (Mahesar et al., 2014). Higher quality oil will have a lower proportion of FFA. Boarfish had the highest levels of polar lipids (PL and GL). Polar lipids are molecules with hydrophobic and hydrophilic ends. They are the major components of cell membranes in eukaryotic animal (PL) and plant (GL) cells. Their chemical structures make them useful natural surfactants that could be used by a range of industries (e.g. petroleum, pharmaceuticals, agrochemicals and cosmetics; Holmberg, 2001; Lourith & Kanlayavattanakul, 2009). Furthermore, research has shown that polar lipids are more easily digested and absorbed and improve digestibility and absorption of TG (Berger, 2014). Therefore, they are becoming a valued fraction in fish oil supplements.
The fatty acid profile of the TG, the principal fraction of the fish oil, varied significantly between species, with herring containing the highest amount of SFA and the lowest relative abundance of PUFA. While blue whiting had significantly higher levels of MUFA and PUFA, boarfish had significantly higher levels of PUFA. Comparing the PUFA and omega-3 content with other marine fish, Atlantic herring had low relative abundance of PUFA and omega-3 fatty acids. Boarfish and blue whiting had higher levels of PUFA and omega-3 fatty acids compared with Atlantic herring, but they had lower levels compared with approximately three-quarters of the fish. Considering total lipid content and fatty acid profile together, herring are a good source of fish oil. Herring has been, and still is, used as a reduction species directed towards fish meal and fish oil production (Neiland, 2007). However, in recent years there has been a revival of a direct human consumption market (Cashion et al., 2017). In terms of maximising food security and sustainability, direct human consumption should be promoted when such a desire exists but extraction of lipid fractions from waste streams may still provide valuable nutrients that could be used in a range of different products. Although still below average compared with the fish from other studies, boarfish and blue whiting contained moderate quantities of total lipids, PUFA and omega-3 fatty acids. The results from this study suggest that boarfish and blue whiting could be as good, if not better sources of fish oils, compared with herring.
Poly-unsaturated fatty acids made up a much larger part (nearly 50%) of the PL fraction compared with the TG fraction in the three fish species. Furthermore, within this fraction herring had significantly higher levels of PUFA and omega-3 fatty acids compared with blue whiting and, blue whiting and boarfish, respectively. In a number of clinical and preclinical trials, PL have been shown to be beneficial for cardiovascular and neurological health (Lee et al., 2010; Richter et al., 2010), as well as for treating symptoms of metabolic syndrome (Banni et al., 2011; Rossmeisl et al., 2012) and inflammatory-related diseases (Bjørndal et al., 2012; Grimstad et al., 2012; Vigerust et al., 2013), sometimes proving more successful than TG equivalents (Rossmeisl et al., 2012). Phospholipid are currently used in nutritional products, in cosmetics and for drug delivery, making them lucrative compounds (the global market was estimated at US$3.04 billion in 2017; Market and Markets Research, 2018). To date, most commercial PL are produced from plant sources (often soy) and from krill (marine copepods). Similar to the results outlined in this study for blue whiting, boarfish and herring, the proportion of the PL fraction in fish oil is often low (Takama et al., 1994) and production of marine PL from fish or fish by-products is limited (Burri et al., 2012). However, there is potential for growth within this market (Burri et al., 2012) and the high values of these compounds provide a strong incentive for product development.
Finally, fish are good sources of micronutrients (e.g. calcium, iron, zinc), superior to other animal-source foods in quality, quantity and bioavailability (Beveridge et al., 2013). They often play an important role providing essential nutrients in poorer countries where diets are dominated by starchy staples (Beveridge et al., 2013; Béné et al., 2015). However, fish are also in intimate contact with their surrounding environment and have a tendency to bioaccumulate heavy metals in their tissues, thus; providing a potential health risk to the consumer. In this study, both the mineral and heavy metal contents of blue whiting, boarfish and herring were investigated.
Boarfish, followed by blue whiting and then by herring, had the highest level of total ash content. This result can be explained by the fact that boarfish have well-armoured, bony bodies with long spines and thick scales, whereas herring, and to a lesser extent blue whiting, are finely boned fish. The morphology of boarfish has caused challenges in the development of the fishery, whereby specialised equipment is required for catching and processing the fish. However, it also makes them an excellent raw material for mineral extraction. All of the minerals analysed, except for potassium and selenium, were found at the highest levels in boarfish. This is the first report of mineral content in boarfish and it highlights this species as an excellent source of calcium, phosphorous and zinc. Comparing the mineral and heavy metal contents of blue whiting and herring detected in this study with other published studies (Martínez-Valverde et al., 2000; Tahvonen et al., 2000), herring had lower levels of all minerals. In contrast, the blue whiting analysed in this study had higher levels of potassium, zinc, sodium and calcium but lower levels of iron, magnesium and phosphorus.
Reviewing the heavy metal content of the three fish species, zinc in boarfish (55.7 mg/kg) was the only heavy metal detected above the specified MAL (30 mg/kg) (Nauen, 1983). However, in contrast, a more recent report from the FAO has recommended an intake of between 4 and 14 mg/day of zinc for an adult man (FAO/WHO, 1998). Zinc in boarfish is found at 5.57 mg/100 g which could be argued to be at an adequate level and suggests that the MAL for zinc in fish should be reassessed. Arsenic was the only other heavy metal that was detected at levels close to the specified MAL. It would be important to closely monitor the content of this metal if these fish were to be processed for mineral streams.
The chemical and nutritional compositional data generated in this study, which include fatty acid profiles as analysed by GC, will benefit the seafood industry. Moreover, they are also important information that could be used for stock quality and prey assessment (Lawson et al., 1998; Hartman & Margraf, 2008). However, it should be noted that the nutrient content of fish is not stable. It varies in relation to many factors including species, sex, biological cycle, body part, season and place of development (Martínez-Valverde et al., 2000). This study focussed on fish in the Northeast Atlantic region. It has shown that three low value pelagic species contain significant amounts of high-quality proteins, lipids and minerals. Future studies would benefit from taking into account catch season, sex and even separating body parts in advance of analysis. Such work would build on these initial data and provide an even greater insight into the proximate composition ranges within these species.
The three species of fish studied here, and especially blue whiting and boarfish, are excellent sources of nutrients that have not previously been exploited to their full potential. The chemical composition of these fish, as outlined in this study, should help to direct future exploitation and guide appropriate product development which will in turn add significant value to these resources for human and animal nutritional applications.