Abbreviations and acronyms: AF, Atrial fibrillation; CA, Catheter ablation; LA, Left atrium; LV, Left ventricle; HR, Hazard ratio; NYHA, New York Heart Association; LVEF, Left ventricular ejection fraction; AAR, Anti-arrhythmic medications; AVN, Atrioventricular node; ARNI, Angiotensin receptor/neprilysin inhibitor; SGLT2, Sodium glucose-like transporter 2 inhibitor; Cryo, Cryoablation; RF, Radiofrequency ablation; LVH, Left ventricular hypertrophy; PV, Pulmonary vein.
Background
Evolving Definition
Atrial fibrillation (AF) is a supraventricular tachyarrhythmia defined by uncoordinated, rapid atrial activation (300–500 beats per minute) resulting in ineffective contraction, with electrocardiographic characteristics including the absence of distinct P waves and presence of irregular atrial activation and R-R intervals [1–3]. Historically, several classification schemes have subdivided AF according to duration (paroxysmal or persistent), assumed mechanism (vagally or sympathetically mediated), association with concurrent organic disease (structural heart disease or valvular disease), and number of associated gene loci (monogenic or polygenic) [2]. Mirroring the structure of the American College of Cardiology (ACC)/American Heart Association (AHA)/Heart Failure Society of America guidelines (and later the 2020 ACC/AHA valve guidelines), recent guidelines recommend classifying recurrent AF according to duration and staging, along a continuum comprising AF, pre-AF, AF, and permanent AF (stages 1–4) (Figure 1) [1].
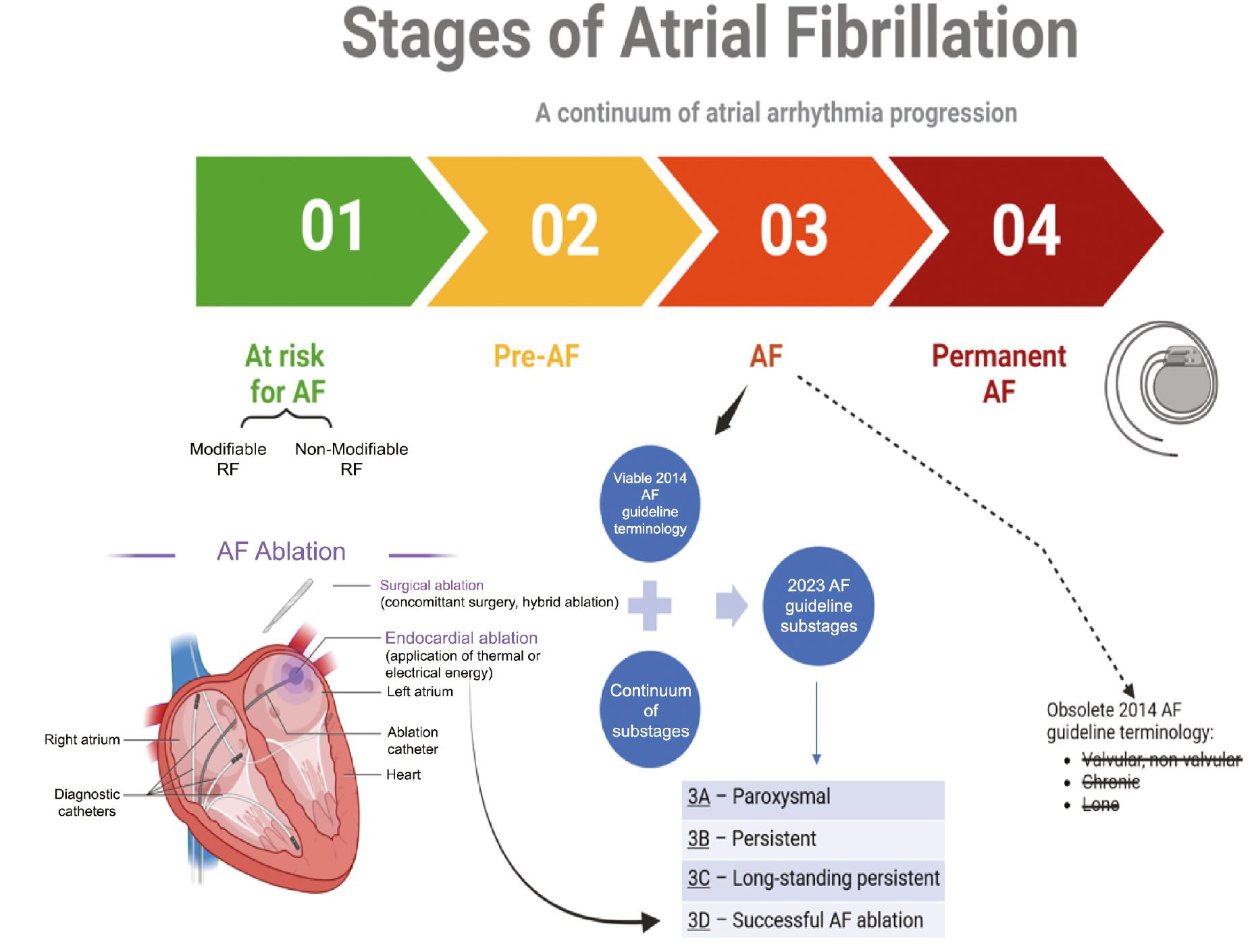
Classification of Atrial Fibrillation (AF) by Stages and Substages According to the 2023 ACC/AHA/HRS Guidelines, which Endorse Considering AF as a Continuum of Stages (Detailed in Table 1).
Similar to heart failure classification, stages 1 and 2 are preclinical, whereas stages 3 and 4 involve clinical AF. Stages 1 and 2 emphasize holistic management by prevention and treatment of modifiable risk factors (stage 1), particularly after the development of structural heart disease before detection of AF (stage 2). Stage 3 is clinical AF that is not yet permanent, which the guidelines divide into four substages (details in Table 1). The guidelines suggest elimination of the listed terminology. Finally, stage 4, permanent AF, involves rate control, often with placement of a pacemaker and AV node ablation [1]. Created with BioRender.
Detailed Description of the Stages and Substages of Atrial Fibrillation (AF) Depicted in Figure 1.
Stage/substage of AF | Description |
---|---|
1 | At risk of AF, with presence of modifiable and nonmodifiable risk factors (listed in detail in Table 2). |
2 | Pre-AF, with evidence of common structural or electrical findings further predisposing patients to AF (e.g., atrial enlargement, frequent atrial ectopy, short bursts of atrial tachycardia, and atrial flutter). Other scenarios of high AF risk include heart failure, valve disease, coronary artery disease, hypertrophic cardiomyopathy, neuromuscular disorders, and thyroid disease. |
3 | AF, subdivided into traditional stages from the 2014 guidelines, with a new substage added for successful AF ablation. Patients may transition among AF substages. |
3A | Paroxysmal AF, which is intermittent and terminates within ≤7 days. |
3B | Persistent AF, which is continuous and sustained for >7 days, and requires intervention. |
3C | Long-standing persistent AF, which continues for >12 months in duration. |
3D | Successful AF ablation, which involves freedom from AF after percutaneous or surgical intervention to eliminate AF. |
4 | Permanent AF, in which no further attempts at rhythm control are made after discussion between patients and clinicians. Permanent AF can be treated simply with rate control or an ablate-and-pace strategy. |
Adapted from the 2023 ACC/AHA/HRS guidelines [1].
The 2024 European Society of Cardiology (ESC)/European Heart Rhythm Association (EHRA)/European Association for Cardiothoracic Surgery (EACTS) guidelines focused on a more descriptive classification of AF, while retaining the temporal labels (i.e., paroxysmal, persistent, and permanent) from prior guidelines [1, 3]. First-diagnosed AF (Table 2) is referred to in both the ACC/AHA/HRS and ESC/EHRA/EACTS guidelines but is not included in the staging for the former guidelines [1, 3]. The 2024 ESC/EHRA/EACTS guidelines strongly emphasize comorbidity and risk factor management, setting precise targets for managing AF-associated conditions and risk factors (e.g., hypertension, heart failure, diabetes, obesity, obstructive sleep apnea, physical activity, and alcohol intake) to ameliorate symptoms and quality of life, decrease AF recurrence and overall AF burden, and slow or prevent AF progression [3, 4].
Selected Updated Definitions and Classifications of Atrial Fibrillation (AF) from the 2024 ESC/EHRA/EACTS Guidelines [3].
Relevant term | Definition |
---|---|
First-diagnosed AF | AF that has not been diagnosed previously, regardless of symptom status, temporal pattern, or duration. |
Recent-onset AF | Accumulating data indicate the value of the term recent-onset AF in decision-making for acute pharmacological or electrical cardioversion of AF. The cut-off time interval to define this entity has not yet been established. |
Trigger-induced AF | New AF episode near a precipitating and potentially reversible factor. |
Early AF | The time since diagnosis that qualifies for early AF is dissociated from any underlying atrial cardiomyopathy and is not well defined, and broadly ranges from 3 to 24 months. The definition of early AF does not necessarily determine early timing of intervention. |
Self-terminating AF | Paroxysmal AF that terminates spontaneously. This definition may be of value in joint decisions on acute rhythm control between patients and healthcare providers. |
Non-self-terminating AF | Atrial fibrillation that does not terminate spontaneously. If needed, termination can be achieved only with an intervention. |
Epidemiology, Incidence, and Prevalence
AF is a complex, multifactorial, progressive disease that increases with age, as well as the burden of cardiovascular disease and other related chronic comorbid conditions (e.g., obesity, pulmonary disease, renal disease, and metabolic disorders) (Table 3). The population of individuals with known AF continues to grow worldwide. According to data from the Framingham Heart Study, it has been estimated that the prevalence of AF increased by more than 4-fold over the last 50 years, possibly due to superior methods of detection, increased incidence, greater survival in patients with cardiovascular conditions predisposing to AF, and greater survival following the onset of AF [6]. In 2020, 50 million individuals were estimated to have AF [1]. Predictions suggest that by 2050, more than 12 million individuals in the United States will be diagnosed with AF [7], and approximately 72 million individuals will be diagnosed across Asia alone [8]. These values are almost certainly an underestimate as one-third of patients with AF are asymptomatic [9]. In addition, wearable devices are used by a minority of the total population and younger individuals are less likely than older individuals to have one or more traditional cardiac risk factors (Table 3) [10].
↑ indicates increased.
Beyond the population level, the risk of AF development is also elevated at the individual level and is modified by socioeconomic, clinical, and genetic factors (Table 3, Figure 2) [1]. Various mechanisms promote the progression of diagnosed paroxysmal AF to persistent AF (Figure 1) in the absence of intervention. In a Canadian registry study in nearly 800 patients with paroxysmal AF, 8.6%, 24.3%, and 36.3% of individuals showed progression to persistent AF within 1, 5, and 10 years, respectively [12].
AF is associated with adverse outcomes, including a 5-fold elevated risk of heart failure, 2.4-fold elevated risk of stroke, 2-fold elevated risk of sudden cardiac death, 1.5-fold elevated risk of cognitive impairment or dementia, 1.5-fold elevated risk of myocardial infarction, 1.5- to 2-fold elevated risk of death, 1.6-fold elevated risk of chronic kidney disease, and 1.3-fold elevated risk of peripheral arterial disease. Treatment and prevention of AF are also associated with potential adverse events [1] (e.g., a 5.7% increase in the risk of gastrointestinal bleeding from appropriate anticoagulation [13]). These observations highlight the importance of weighing risks and benefits in reviewing treatment options with patients.
Risk Factors for Atrial Fibrillation
Because AF is a heterogeneous disease, paroxysmal atrial fibrillation can markedly differ in clinical presentation among patients (for example, a patient with a pulmonary vein trigger without apparent heart disease, vs. a patient with structural heart disease, heart failure, or long-standing hypertension with a high burden of atrial fibrosis). Atrial fibrillation is not an isolated arrhythmic condition, nor is it caused by a single condition. The risk factors of AF are multifactorial and summarized in Table 3. These patient risk factors interact with clinical and procedural factors to determine the success of catheter ablation (Figure 2).
Pathophysiology of Atrial Fibrillation
The simplified pathophysiology of atrial fibrillation involves a trigger initiating the arrhythmia and a substrate responsible for the maintenance of AF [14]. Triggers are foci within or outside the pulmonary veins; approximately 70%–90% are peri- or intra-pulmonary vein triggers [1, 15, 16]. The electrical abnormalities observed in electrocardiograms during AF represent a shared phenotype and nexus of complex pathophysiologic features, including atrial structural abnormalities (e.g., dilation, ischemia, infarction, and fibrosis), atrial electrical abnormalities (e.g., tissue heterogeneity, decreased conduction, increased automaticity, decreased action potential duration/refractoriness, and abnormal intracellular calcium handling), autonomic neural dysregulation, metabolic influences, environmental influences, and genetic influences [1, 17]. These features can result in a vicious cycle of electroanatomic derangements leading to atrial cardiomyopathy and progression toward permanent atrial fibrillation. Collectively, these various electrical and microscopic structural changes converge in stabilizing and promoting AF by creating permanent changes to the underlying myocardial substrate required for maintenance of AF.
Drug Trials: Rate vs. Rhythm Control
A summary of early trials investigating rate control vs. rhythm control is provided in Table 4. Death, disabling stroke, bleeding, cardiac arrest, heart failure, cardiovascular hospitalization, and symptoms were primary or secondary outcomes in these trials.
Early Trials Investigating Medical Therapies of Rhythm Control vs. Rate Control in Patients with Atrial Fibrillation [18, 19].
Trial (RCT) | PIAF 2000 | RACE 2002 | AFFIRM 2002 | STAF 2003 | RACE-HF 2005 | AFFIRM-HF 2007 | AF-CHF 2008 | HOT CAFÉ- 2009 |
---|---|---|---|---|---|---|---|---|
Sample size | 252 | 522 | 4060 | 200 | 261 | 788 | 1376 | 61 |
Mean age (years) | 60.5 | 68 | 69.7 | 65.8 | 69 ± 9 | 70 ± 9 | 67 ± 11 | 72.4 ± 7.1 |
Female (%) | 27 | 36.6 | 39.3 | 36.5 | 35 | 25 | 18 | 16 |
AF pattern | Persistent, symptomatic AF | Recurrent persistent AF or atrial flutter | AF likely to be recurrent | Persistent AF with moderate to high risk of recurrence | Persistent AF | Paroxysmal, persistent AF | Paroxysmal, persistent AF | Persistent AF |
AF duration | Rate: 118 days (mean) Rhythm: 103 days (mean) | Rate: 333 days (median) Rhythm: 309 days (median) | N/A: 69.2% had AF ≥ 2 days for qualifying episode | Rate: 10.4 months (mean) Rhythm: 13.7 months (mean) | 17.7 ± 59 | 44% > 6 month AF duration | 14 ± 6.5 | |
Cardiac function | NYHA I–III | LVEF 54.7% | NYHA II–III | NYHA II or III | LVEF < 50% | NYHA II–IV or LVEF ≤ 35% | NYHA II or III | |
LA diameter (mm) | Rate: 46 ± 7 | 45 ± 7 | Enlarged in 65.7% | 46 ± 6 | 46 ± 7 | 49 ± 7 | 47 ± 10 | |
Rhythm control | Electrical cardioversion and AAR | Electrical cardioversion and AAR | Electrical cardioversion and AAR | Electrical cardioversion and AAR | Electrical cardioversion and AAR | Electrical cardioversion and AAR | Electrical cardioversion and AAR | Electrical cardioversion and AAR |
Rate control | Primarily diltiazem; other AVN blockers at physicians discretion | Beta-blocker, calcium channel blocker, digitalis | Beta-blocker, calcium channel blocker, digitalis | Beta-blocker, calcium channel blocker, digitalis | Beta-blocker, calcium channel blocker, digitalis | Beta-blocker, calcium channel blocker, digitalis | Beta-blocker, digitalis, AV node ablation + PM (if indicated) | Beta-blocker, digitalis |
Primary outcome | Improvements in AF related symptoms | Composite of cardiovascular death, heart failure, thromboembolic complications, bleeding, pacemaker implantation, severe adverse effects of antiarrhythmic drugs | Overall mortality | Composite of death, cardiopulmonary resuscitation, cerebrovascular event, systemic embolism | Composite cardiovascular death, HF, embolism, bleeding | All-cause mortality | Cardiovascular death | Quality of life |
Discussion in main text.
AF, atrial fibrillation; LA, left atrium; N/A, not applicable; NYHA, New York Heart Association; LVEF, left ventricular ejection fraction; AAR, anti-arrhythmic medications; AVN, atrioventricular node; HF, heart failure.
Drug trials in the early 2000s compared rate control against rhythm control through cardioversion and antiarrhythmic drugs. Neither the AFFIRM nor the HOT CAFÉ trial demonstrated decreases in all-cause mortality or stroke rates with rhythm therapy [1, 20, 21]. A meta-analysis of randomized trials and observational studies confirmed no decreases in all-cause mortality, cardiac mortality, or stroke rates with rhythm control [1, 22]. Those early drug trials concluded that drug-treated rhythm control was not superior to rate control. These observations led to the AHA/ACC/HRS 2014 guidelines suggesting that rate control is safe and preferred in less symptomatic patients, and that rhythm control, with follow-up (i.e., monitoring drug adverse effects and drug-drug interactions), is preferred in more symptomatic patients [23]. These recommendations were concordant with the worldwide guidelines [24–26], although nuances existed in interpretation. The Canadian and Australian/New Zealand guidelines suggested rhythm control in paroxysmal AF, while recommending rate control for persistent AF, particularly long-standing AF [25, 26]. Taiwanese guidelines recommended rate control for a low symptom burden, through an individualized approach, given the preliminary but not robust data supporting rhythm control at that time [24]. The guidelines from both AHA/ACC/HRS and ESC/EHRA/EACTS endorsed shared decision-making for up-front rate vs. rhythm control, while acknowledging that most patients require a combination aproach [1, 3]. However, the rhythm control interventions in these investigations in the early 2000s did not include CA as a therapeutic option. In the AFFIRM study, the crossover rate at 5 years was significantly greater in the rhythm control group (38%) than the rate control group (15%), mainly because of uncontrolled symptoms [20]. The authors noted that antiarrhythmic drugs frequently failed [20] In the rate control group, 34% of patients who originally crossed over to rhythm control had crossed back into their original group by the end of the study.
Early Ablation vs. Drug Trials for Rhythm Control
As evidence accrued, CA became a more prevalent therapeutic option for rhythm control. A meta-analysis in 2009 identified 63 studies (nine of which were RCTs) examining CA and 34 studies examining AAR. The success rates (the absence of recurrent arrhythmia during the follow-up period) with CA for single and multiple procedures were 57% and 71%, respectively (and this rate increased to 77% with adjunctive AAR). The treatment success rate with AAR was 52%. The early ablation trials demonstrated that CA for the treatment of AF was associated with higher efficacy and fewer adverse events than AAR [27]. Importantly, at least one AAR attempt had failed in most participants before trial entry.
Another meta-analysis was performed in 2015, with a focus on quality of life, morbidity, and mortality [28]. That analysis included 12 RCTs completed between 2003 to 2014 and involving a total of 1707 patients. Compared to AAR medications, CA was associated with greater improvements in the 436-item Short Form Health Survey areas and the symptom frequency score from baseline to 3 months; however, these improvements were not sustained over longer follow-up periods. The overall morbidities, including TIAs and stroke, were low and did not differ between the use of CA and drugs. Moreover, mortality rates did not differ between treatment arms. These results led to consistent recommendations across international guidelines until 2017. CA ablation for AF was primarily for symptomatic patients. CA was a class IIa recommendation for paroxysmal AF and IIb recommendation for persistent AF. CA was a class I recommendation for patients with paroxysmal AF after failure of one or more AAR attempts, drug intolerance, or a preference for CA [2, 23–26].
While there is clear evidence for improved rhythm control with CA, consistent evidence of benefit in critical clinical outcomes such as survival or stroke risk is lacking. This discordance might be explained by the factors shown in Figure 2. Advances in the technology and tools used during ablation have made the procedure safer and more effective. Additionally, ablation techniques have advanced, and wide-area circumferential ablation to isolate the pulmonary veins is now an international standard [1–3, 24, 29, 30], instead of prior techniques such as focal or segmental ablations. Concurrently, the demographics of patients with AF are changing with advances in overall health care. Currently, patients with AF are generally older and have more co-existing medical illnesses than the patients presenting for ablation two decades ago [31–33].
Another plausible explanation for the apparent discordance between better rhythm control by CA and the absence of clinical benefits regarding survival or stroke might be the timing of CA. When CA is performed, the intervention might be “too little, too late,” given that earlier guidelines required most patients to experience drug failure in a trial before undergoing CA. During that period, a patient’s atria might have already undergone negative structural remodeling (Figure 2). Clinical trials began to examine this hypothesis by using CA as the first-line therapy (in the absence of failure of at least one attempt of AAR) for rhythm control.
Atrial Fibrillation Ablation as a First-Line Therapy
Should CA be the first-line therapy for patients with AF? Studies (RAAFT-1, MANTRA-PAF, and RAAFT-2) before 2010 indicated that first-line CA was more effective than AAR in preventing AF recurrence, and the crossover rate from AAR to CA was high. However, procedural techniques and study endpoints were not standardized [34]. As technology and ablation techniques matured, additional studies were performed (CRYO-FIRST, STOP-AF, and EARLY-AF). However, the sample sizes in these studies were not robust, leaving questions regarding the effects of earlier or first-line CA on clinical “hard endpoints” (survival, heart failure, or stroke) unanswered.
CA as a first-line therapy affecting patient outcomes has been evaluated in several recent meta-analyses (summarized in Table 5) [34–38]. Those studies indicated a significant decrease in any recurrence or symptomatic recurrence of atrial arrhythmias with CA, in contrast to AAR. Studies using either cryoablation or radiofrequency ablation were included in these meta-analyses. Major adverse events, including death (0.5%), major bleeding, cardiac tamponade (1.3%), severe PV stenosis (0.3%), atrioesophageal fistula (0%), and stroke (0.8%), were low with CA [36]. Moreover, among patients randomized to CA in comparison to AAR, CA was associated with less healthcare utilization and 79% less crossover during follow-up [35].
Relative Risk Reduction Ratios in Meta-Analyses Comparing Catheter Ablation and Antiarrhythmic Medication as First-Line Strategies for Treatment of Atrial Fibrillation. a
Any recurrence of atrial tachyarrhythmia | Symptomatic recurrence of atrial tachyarrhythmia | Severe adverse effects | Healthcare utilization | |
---|---|---|---|---|
Imberti (2021) [35] | 0.64 (0.51–0.80) | 0.53 (0.35–0.79) | 0.87 (0.58–1.30) | 0.65 (0.48–0.89) |
Saglietto (2021) [36] | 0.58 (0.46–0.72) | 0.46 (0.27–0.79) | No significant increase | |
Ullah (2023) [37] | 0.54 (0.39–0.75) | 2.65 (0.6–11.5) | ||
Khieri (2021) [38] | 0.57 (0.43–0.75) | 0.45 (0.25–0.80) | 1.11 (0.76–1.61) | 0.33 (0.21–0.52) |
Andrade (2023) [34] | 0.69 (0.59–0.82) | 0.56 (0.36–0.87) | 0.74 (0.35–1.56) | 0.71 (0.56–0.92) |
aCryo, cryoablation; RF, radiofrequency ablation; AAR, antiarrhythmic drugs; CA, catheter ablation.
Most randomized controlled trials in these analyses included patients with paroxysmal AF [36, 37]. In the RAAFT-1 trial, only 3% and 5% of individuals had persistent AF in the CA and AAR groups, respectively [39]. In the EARLY-AF trial, only 4.5% and 6% of patients receiving CA vs. AAR therapy had persistent AF. One RCT focused on persistent AF (>7 days, < 12 months) in individuals < 70 years of age and found a 26.6% absolute risk reduction (95% CI 10–43.3; P = 0.002) in the rates of any AF recurrence with CA vs. AAR [40]. Another analysis indicated that the rates of maintenance of sinus rhythm after CA (with PVI alone) were more variable but comparable (56%–87%) to the known rates in patients with paroxysmal AF (60%–80%) [41]. These findings suggested that first-line ablation would be a reasonable strategy in individuals with paroxysmal and persistent AF.
Ablation in Selected Patients
EAST-AF NET
The most robust trial testing early rhythm control vs. usual care was the Early Rhythm-Control Therapy in Patients with Atrial Fibrillation (EAST-AFNET 4) trial. This randomized parallel-group, open-label assessment trial was conducted in 135 countries, and enrolled 2789 patients with AF diagnosed within the prior 12 months (median time from diagnosis 36 days) [42]. The intervention was rhythm control with an antiarrhythmic drug or CA. Usual care comprised rate control without rhythm control unless symptoms temporarily required antiarrhythmic treatment for stabilization. The primary outcome was a composite measure (death from cardiovascular causes, stroke, or hospitalization [from heart failure exacerbation or acute coronary syndrome]) and the number of nights spent in the hospital per year. Secondary outcomes included the presence or absence of symptoms, left ventricular function, LVEF change, and various scores. The trial was stopped early for efficacy at the third interim analysis after 5.1 years of follow-up. A decrease in the composite primary outcome (HR 0.79; 96% confidence interval 0.66–0.94; P = 0.005) with early rhythm control was observed across predefined subgroups, including asymptomatic patients, obese patients, and patients with or without heart failure. The median number of nights spent in the hospital, primary safety outcomes, symptoms, and left ventricular function at 2 years, did not significantly differ between groups. Approximately 30% of the patients in each group had stable heart failure, and the mean CHA2DS2-VASc score was approximately 3.3 in both groups [42].
At 2 years, only 65.1% of patients were still receiving rhythm control medical therapy, representing a decrease with respect to the 94.8% at the time of randomization to rhythm therapy [42]. The decrease in the AAR use might be partly explained by 19.4% of patients having received CA during the trial. In addition, contemporaneous society guidance on the safe use of AAR for rhythm control might also have decreased adverse events associated with AAR use [23, 43]. Most importantly, the early rhythm control was likely to have slowed AF progression and associated comorbidities.
The EAST-AFNET 4 trial demonstrated that early rhythm control had positive effects beyond maintenance of sinus rhythm on a composite endpoint including cardiovascular death, stroke, and heart failure hospitalization. The “early” rhythm control was reflected by the median of 36 days from AF diagnosis [42]. The study included patients with two or more cardiovascular risk factors, such as age > 65 years, female sex, heart failure, hypertension, diabetes, coronary artery disease, chronic kidney disease stage III or IV, and LVH with septal thickness > 15 mm. The mean age of the study cohorts was 70.3 years. The study cohorts also included asymptomatic patients (30.4%), patients with first documented AF (38%), and patients with persistent AF (64%) [42]. Although the EAST-AFNET 4 trial was a rhythm control trial not limited to CA, the outcomes provided compelling evidence supporting the “too little, too late” hypothesis. These findings, combined with the large body of literature demonstrating CA’s superiority to AAR for sustainable rhythm control, suggested that early CA is warranted in selected patients [42].
CABANA: Effects of Ablation vs. Drug Therapy on Death, Disabling Stroke, Serious Bleeding, and Cardiac Arrest
The Catheter Ablation vs. Antiarrhythmic Drug Therapy (CABANA) trial was an open-label, multicenter, multi-national randomized trial in 2204 adults with documented AF for at least 1 hour and evidence of symptoms within the preceding 6 months, who were receiving active therapy (either rate or rhythm control) [33]. The median age was 68 years, and the CHA2DS2-VASc score was 3. The patients were predominantly men (60.8%) and patients with persistent AF (57.1%). The primary outcome, a composite of death, disabling stroke, serious bleeding, or cardiac arrest, occurred in 8.0% of the CA group vs. 9.2% of the drug therapy group, representing a nonsignificant difference (HR 0.83 [CI 0.6–1.21), P = 0.3) [33]. Regarding the secondary endpoints, no difference was observed in all-cause mortality (HR is 0.85, P = 0.38); however, decreases in death or cardiovascular hospitalizations (0.83 [95% CI, 0.74–0.93]; P = 0.001) and AF recurrence (50% vs. 69%; HR 0.52 [95% CI, 0.45–0.60]; P < 0.001) were observed after 4 years of follow-up. Adverse effects associated with ablation were low, most frequently hematoma (2.3%) and pericardial effusion not requiring intervention (2.2%) [33].
The intention-to-treat analysis was confounded by post-randomization crossover and patient withdrawal. The mortality of the trial was one-third less than the predicted mortality rate for the power calculation, thus resulting in an underpowered the trial sample size. Evaluation of the results according to the on-treatment analysis indicated significantly lower attainment of the primary endpoint with CA than drug therapy (HR 0.73, 95% CI 0.54–0.99; P = 0.046) at 12 months [33].
Subgroup analyses showed that CA was associated with a 17% reduction in mortality or CV hospitalization (P = 0.01), a reduction in AF recurrence (P < 0.001), and benefits in primarily younger patients (<65 years old) [33]. Patients with NYHA class II–IV symptoms showed all-cause mortality benefits following CA [33]. However, these subgroup analyses are hypothesis-generating. Mortality benefits observed in younger patients and patients with heart failure were promising and warrant further investigation. These observations suggest that CA is not a “one size fits all” treatment for all patients with AF. Beyond better maintenance of sinus rhythm by CA, emerging data support long-term mortality and morbidity benefits of this treatment in selected populations, such as younger patients, and patients with HF in the presence of AF [42]. These findings are consistent with a prior meta-analysis showing an all-cause mortality benefit of 31% (0.69, 0.54–0.88; P = 0.003) driven by patients with AF and heart failure with reduced EF (0.52, 0.35–0.76; P = 0.0009), reducing CV hospitalizations (0.56, 0.39–0.91; P = 0.002) and recurrences of atrial arrhythmias (0.42, 0.33–0.53; P < 0.00001) [45].
Heart Failure Population
Since 2016, CA has consistently demonstrated benefit in the population with HF [46]. Although many studies have shown improvements in parameters such as EF or percentage of time in sinus rhythm, we will discuss the studies indicating decreased mortality.
CASTLE-AF, in 2018, was a randomized study investigating CA vs. medical therapy in 363 patients with paroxysmal or persistent AF who experienced AAR failure, unacceptable side effects, or were unwilling to receive AAR. Additional inclusion criteria were LVEF ≤ 35%, the presence of an ICD, and NYHA class II–IV symptoms, and ICD [47]. The primary endpoint was a composite of all-cause mortality or heart failure hospitalization. After 37.8 months, CA resulted in a significant decrease in the primary endpoint (20.5 vs. 44.6%, HR 0.62; 95% CI 0.43–0.87; P = 0.007). Individually, all-cause mortality (13.4 vs. 25%, HR 0.53; 95% CI, 0.3–0.86; P = 0.01), cardiovascular mortality (11.2 vs. 22.3%, HR 0.49; 95% CI 0.29–0.84; P = 0.009), and HF hospitalization (20.7 vs. 35.9%, HR 0.56; 95% CI =, 0.37–0.83; P = 0.004) were significantly decreased [47]. LVEF also improved a median of 8.0% over 60 months, with a greater increase in individuals with persistent (10.1%) vs. paroxysmal AF (7.3%) [47]. The 6-minute walk distance significantly improved at 12 months (41 m) with CA.
The CABANA HF substudy investigated 778 patients from the original 2019 study [33] with clinical HF (80% HFpEF), who were randomized to receive CA (378) or medical therapy (400) [44]. CA was associated with a decrease in the composite of MACE (HR 0.64; 95% CI, 0.41–0.99) and an improvement in all-cause mortality (HR 0.57; 95% CI, 0.33–0.96) [44]. The burden of AF was reduced in the post-blanking period (HR 0.56; 95% CI, 0.42–0.74) with improvement in quality of life parameters [44]. In the CABANA HF substudy, the first RCT assessing CA vs. drug therapy in a population with HFpEF, CA had superior results at 12 months in patients with both paroxysmal and persistent AF [44], thus indicating that the benefits of CA in HF transcended EF categories.
The CASTLE HTx study published in 2023 was a single-center, open-label, randomized trial based in Germany, in 194 patients with symptomatic atrial fibrillation and end-stage heart failure (EF ≤ 35%, ICD, diminished 6MWT, referred for advanced therapies, etc.) [31]. The primary endpoint was a composite of death from any cause, implantation of a left ventricular assist device, or urgent heart transplantation. The follow up duration was 18 months. CA was performed in 84% of the ablation group and 16% of the medical therapy group. CA resulted in lower attainment of the primary endpoint (8%) than medical therapy (30%) (HR 0.24; 95% CI 0.11–0.52; P < 0.001) [31]. CA also was associated with lower all-cause mortality (6%) than medical therapy (20%) (HR 0.29; 95% CI, 0.12–0.72) [31].
Several meta-analyses assessed various CA parameters in patients with HF. The results for all-cause mortality are shown in Tables 6 and 7.
Pooled Analysis of All-Cause Mortality after Catheter Ablation Rhythm Control vs. Medical Therapy [48].
Study/subgroup | Ablation rhythm control events | Ablation rhythm control total | Medical therapy events | Medical therapy total | Weight | OR |
---|---|---|---|---|---|---|
ARC-HF 2013 | 1 | 26 | 0 | 26 | 1.3% | 3.12 (0.12, 80.12) |
CAMTAF 2014 | 0 | 26 | 1 | 24 | 1.3% | 0.30 (0.01, 7.61) |
AATAAC 2016 | 8 | 102 | 18 | 101 | 17% | 0.39 (0.16, 0.95) |
CASTLE-AF 2018 | 24 | 179 | 46 | 184 | 44.7% | 0.46 (0.27, 0.80) |
CABANA-HF-subgroup 2019 | 21 | 174 | 29 | 163 | 35.9% | 0.63 (0.35, 1.16) |
Total | 54 | 507 | 94 | 498 | 100% | 0.51 (0.36, 0.74) |
Pooled Analysis of Re-Hospitalization after Catheter Ablation Rhythm Control vs. Medical Therapy [48].
Study/subgroup | Ablation rhythm control events | Ablation rhythm control total | Medical therapy events | Medical therapy total | Weight | OR |
---|---|---|---|---|---|---|
AATAAC 2016 | 32 | 102 | 58 | 101 | 42.4$% | 0.34 (0.19, 0.60) |
CAMERA-MRI 2017 | 0 | 33 | 4 | 33 | 3.2% | 0.1 (0.01, 1.89) |
CASTLE-AF 2018 | 64 | 179 | 89 | 184 | 54.4% | 0.59 (0.39, 0.90) |
Total | 54 | 507 | 94 | 498 | 100% | 0.51 (0.36, 0.74) |
This combined analysis indicated that CA was associated with significantly lower all-cause mortality (Table 6) and less rehospitalization (Table 7) than medical therapy. In addition, CA was associated with significantly improved EF (6.8%), less AF/AT recurrence (96% relative risk reduction), and improved quality of life (9.1 point decrease in MLHFQ score) (analyzed, but not depicted) [48]. Composite adverse events showed a non-significant trend toward decreasing with CA (0.66 [0.33, 1.34]) [48]. These results underscore the important role of CA in restoring sinus rhythm and the importance of preserving and restoring normal atrial physiology toward overall global cardiac function, as well as the benefits and safety of CA in functional outcomes [48].
Specific Risk Factors Favoring First-Line Ablation
The new guidelines and consensus statements highlight specific populations benefitting from CA in early stages of disease. In athletes who develop AF, CA with PVI is a reasonable strategy for rhythm control, because of its effectiveness, low risk of detrimental effects on exercise capacity, and significant improvements in quality of life. Recent AHA/ACC/HRS guidelines and the HRS expert consensus statement (ECS) generally favor first-line ablation in athletes, but differ in the class of recommendation (guidelines [1]: II; ECS [5]: I) and level of evidence (gudelines [1]: B; ECS [5]: A for quality of life, B for exercise performance). Similarly, patients with primary arrhythmia syndromes or inherited cardiomyopathies with AF associated with elevated risk of adverse events from AAR (e.g., amiodarone) or limited options because of contraindication (e.g., classes IA or III in long QT syndrome, class IC in Brugada), also benefit from early CA [3]. Additionally, patients developing unexplained AF before 30 years of age may benefit from an EP study to identify and ablate concurrent supraventricular tachycardias triggering AF [1, 3]. Finally, CA in patients with AF and symptomatic bradycardia or sinus pauses after AF termination may be reasonable to avoid pacemaker implantation [1, 3, 30].
Certain risk factors have been demonstrated to predispose specific populations with AF to delayed access to CA, including female sex, relatively low education and health literacy, urban/rural setting, disability, and social isolation [3, 49]. These factors may be considered in determining when to discuss CA as a tool for AF management in patients who might not have future access.
Current Guidelines: Ablation as a First-Line Therapy
In this review, we aim to provide a succinct synthesis of a large body of evidence to explain the most up to date evidence of CA as a first-line therapy (Table 8).
Summary of Recommendations for CA as a First-Line Therapy from the 2023 AHA/ACC/HRS Guidelines, 2024 ESC AF Guidelines, 2024 EHRA/HRS/APHRS/LAHRS Consensus Statement, and 2024 HRS Expert Consensus Statement on Arrhythmias in the Athlete: Evaluation, Treatment, and Return to Play. a
Societies represented | Class of evidence | Level of evidence | Recommendations |
---|---|---|---|
AHA/ACC/HRS, ESC/EHRA/EACTS | I | A | In selected patients (generally younger patients with few comorbidities, per AHA/ACC/HRS) with symptomatic paroxysmal AF in whom rhythm control is desired, catheter ablation is useful as a first-line therapy to improve symptoms and decrease progression to persistent AF. |
AHA/ACC/HRS, ESC/EHRA/EACTS | IIa | B-R | In patients (other than younger patients with few comorbidities, per AHA/ACC/HRS) with symptomatic paroxysmal or persistent AF who are being managed with a rhythm-control strategy, catheter ablation as a first-line therapy can be useful to improve symptoms. |
AHA/ACC/HRS, ESC/EHRA/EACTS, EHRA/HRS/APHRS/LAHRS | I | B-NR | In patients presenting with a new diagnosis of HFrEF and AF, arrhythmia-induced cardiomyopathy should be suspected, and an early and aggressive approach to AF rhythm control is recommended. |
AHA/ACC/HRS, ESC/EHRA/EACTS, EHRA/HRS/APHRS/LAHRS | I | A B (ESC) | In appropriate patients with AF and HFrEF who are receiving GDMT, and have reasonable expectation of procedural benefit (Figure 24), catheter ablation is beneficial to improve symptoms, QOL, ventricular function, and cardiovascular outcomes. |
ESC/EHRA/EACTS | IIa | B | AF catheter ablation should be considered in selected patients with AF with HFrEF to decrease HF hospitalization and prolong survival. |
HRS Expert consensus statement on arrhythmias in the athlete | I | B-R | In athletes with symptomatic AF, catheter ablation is recommended as a first-line therapy, or if antiarrhythmic drugs are contraindicated or poorly tolerated. |
AHA/ACC/HRS | I | A | In patients undergoing ablation for AF, PVI is recommended as the primary lesion set for all patients unless a different specific trigger is identified. |
ESC/EHRA/EACTS, EHRA/HRS/APHRS/LAHRS | I | A | Catheter ablation is recommended as a first-line option within a shared decision-making rhythm control strategy in patients with paroxysmal AF, to decrease symptoms, recurrence, and progression of AF. |
AHA/ACC/HRS, ESC/EHRA/EACTS | IIb | B-NR C (ESC) | Catheter ablation may be considered as a first-line option within a shared decision-making rhythm control strategy in selected patients with persistent AF, to decrease symptoms, recurrence, and progression of AF. |
aEHRA, European Heart Rhythm Association; APHRS, Asian Pacific Heart Rhythm Society; LAHRS, Latin American Heart Rhythm Society; ESC, European Society of Cardiology; AF, Atrial Fibrillation; EHRA, European Heart Rhythm Association; EACTS, European Association for Cardio-Thoracic Surgery [1, 3, 5, 30].
The recent AHA/ACC/HRS guidelines elevated CA as a first-line therapy in selected, young, relatively healthy individuals with symptomatic, paroxysmal AF with a desire for rhythm control [1]. CA of AF is now an IA recommendation to improve symptoms and decrease progression to persistent AF [1]. The guidelines take a more cautious approach toward older individuals with more comorbidities, assigning an IIA recommendation to first-line ablation and advocating for ablation as a helpful therapeutic option for symptomatic improvement in paroxysmal or persistent AF [1]. The new ESC/EHRA/EACTS guidelines assign a class I recommendation for first-line CA in all patients with paroxysmal AF. Both guidelines emphasize careful selection of asymptomatic patients [1, 3]. The ESC/EHRA/EACTS guidelines assign a class I recommendation to surgical ablation in individuals undergoing mitral valve surgery who are candidates for rhythm control [3], whereas the ACC/AHA/HRS guidelines assign this population a IIA recommendation [1]. The ESC/EHRA/EACTS guidelines assign persistent patients with AF a class IIb recommendation for first-line CA, whereas ACC/AHA/HRS assign this group a IIa recommendation [1, 3]. Both guidelines are in agreement that in selected patients with asymptomatic or minimally symptomatic AF, CA may be useful (class IIb) for decreasing the progression of AF and its associated complications [1, 3].
The discussion of slowing AF progression by CA featured prominently in the guidelines, given that notable studies in the past decade have consistently shown that without intervention, AF continues to progress to a higher symptom burden associated with poorer clinical outcomes [1, 12, 50–55].
The recommendations for CA as a first-line therapy in the most recent AHA/ACC/HRS and ESC/EHRA/EACTS guidelines are summarized in Table 8. These recommendations are in agreement with the recently released expert consensus statement by heart rhythm societies worldwide [1, 3, 30].
Limitations of Cardiac Ablation
Although the benefits of CA of AF have been extensively discussed, the limitations of the procedure are also worthy of discussion. These limitations encompass procedural complications and recurrence in certain populations.
Common complications include vascular access bleeding (1%–4%) and asymptomatic acute cerebral lesions (5%–30%) [30]. Rare complications (<1% prevalence) include permanent phrenic nerve palsy, severe pulmonary vein stenosis, cardiac tamponade, periprocedural thromboembolic event, atrioesophageal fistula (AEF), and death [30]. With the advent of pulsed field ablation, some of the more feared complications (AEF, phrenic nerve palsy, and PV stenosis) observed with thermal ablation were not observed in a recent study of over 17,000 patients [56]. Adoption of pulsed field ablation (PFA) has been rapid, and some reports have estimated that this method would account for as many as 40% of ablations in mainland China by the end of 2024 and 50% of those in the US market by 2028 [56, 57]. Notable PFA-related adverse events include (reversible) epicardial coronary arterial spasm (0.14%) and hemolysis causing acute renal insufficiency requiring hemodialysis (0.03%) [56]. Additionally, CA has limited effectiveness certain populations, including patients with long-standing persistent AF, severe atrial myopathy (dilation/fibrosis), certain cardiomyopathies (e.g., hypertrophy cardiomyopathy [58] and amyloidosis) [1, 3, 30], and significant ventricular scarring on CMR [1]. AF is also usually poorly hemodynamically tolerated in these populations. Therefore, adequate informed consent and shared decision-making with the patient should guide determination of the best strategy among CA of AF (with or without antiarrhythmic therapy) and an ablate-and-pace rate control strategy.
Rienstra and colleagues have emphasized that although CA is a potential first-line therapy for rhythm control, not every patient with paroxysmal AF should undergo CA, given their observation that 25% of patients presenting with first-time AF showed either no recurrence or a single recurrence of AF over a 3 year period of continuous monitoring [4]. Therefore holistic management of each patient is paramount, to address risk factors, comorbidities, and symptoms, and highlight stroke prevention, with dynamic reassessment. These pathways (e.g., AAAA/SOS/HEAD-2-TOES, AF-CARE) are discussed in detail in recent guidelines [1, 4, 30].
Old and New Technologies Complementing Catheter Ablation of Atrial Fibrillation
Aggressive risk factor management is complementary to CA and a critical component of post ablation management as well as a central focus of the new guidelines and society recommendations [1, 3, 4]. Initial trials suggest that aggressive hypertension management might be important in preventing post ablation recurrence of AF. A meta-analysis of 16 observational studies has revealed a significant 13% greater post ablation AF recurrence for every 5 unit increase body mass index [2]. This finding was confirmed in the ARREST-AF study (in which procedure arrhythmia-free survival markedly improved with risk factor modification (16%) vs. controls (42%) [59] and the LEGACY study (in which weight loss ≥ 10% resulted in a 6-fold improvement in arrhythmia free survival (95% CI, 3.4–10.3; P < 0.001) [60]. Management of sleep apnea is also important, as OSA is associated with a more complex atrial substrate, more frequent non-pulmonary vein triggers, and a higher post-ablation arrhythmia burden independent of obesity and LA size [2]. Moderate alcohol consumption (three or fewer drinks/week), smoking cessation, optimization of exercise (≥210 minutes/week), and pharmacologic management of diabetes care (e.g., metformin or SGLT2 inhibitors) have also been endorsed in recent guidelines.
Because CA is an important tool for keeping patients in sinus rhythm, this toolbox is growing with the new ablation modalities described below.
High Power Short Duration Ablation Lesions
High power short duration ablation has been applied to CA to produce continuous, transmural lesions, while limiting collateral damage, primarily esophageal damage. This technique has shown initial promise with higher first-pass pulmonary vein isolation and a decreased risk of reconnection [61]. Very high power and short duration have also achieved promising results (82% freedom from arrhythmia recurrence at 12 months and 92.1% freedom from repeat ablation) [62]. Despite initial concerns about silent cerebral emboli (SCE), recent trials have shown SCE to be not significantly increased with high-power, short-duration lesions.
Pulsed Field Ablation
PFA is a novel ablation modality that applies ultra-rapid, high-voltage microsecond electrical pulses to the endocardium, thus causing irreversible electroporation of the targeted myocardial tissue with resultant cell death. In preclinical and clinical studies, this method has shown some degree of selectivity while avoiding damage to neighboring structures. The initial results (PULSED AF) showed modest effectiveness at 1 year in both paroxysmal AF (66.2%; 95% CI, 57.9%–73.2%) and persistent AF (55.1; 95% CI, 46.7–62.7), with an acceptable safety of 0.7% in both cohorts [63]. The ADVENT trial comparing PFA to thermal ablation has demonstrated non-inferiority regarding freedom from arrhythmia (73% PFA, 71.3% thermal ablation) and safety endpoints (2.1% PFA, 1.5% thermal ablation) at 1 year [64]. Most recently, the MANIFEST-17K study has established the safety of PFA across 116 centers in more than 17,000 patients, with no cases of AEF, PV stenosis, or phrenic paralysis and low rates of tamponade (0.36%), TIA/stroke (0.12%), or death (0.03%) [56]. In recent months, four platforms have been approved by the FDA [65–68]. More than 60 studies are pre-enrollment (19), are actively recruiting (41), or have completed enrollment (15) [69].
Current Gaps in Knowledge and Directions for Future Research
Despite substantial advancements in the CA of AF, important knowledge gaps remain [1, 3, 5, 30, 70–72]:
More long-term (>1 year), high-quality evidence regarding the effectiveness of ablating subclinical (truly asymptomatic) AF is needed.
With the seemingly improved safety of AF CA, is there an age beyond which the risks of CA exceed the benefits? Is age or frailty a better marker for answering this question?
What is the optimal “blanking period” after AF ablation? Should this period differ by baseline AF burden and underlying risk factors?
What is the optimal time to refrain from intense exercise after ablation in athletes?
What is the optimal definition of AF burden to define recurrence?
What is the effectiveness of CA in older patients with AF?
What is the durability of PFA ablation lesions?
What are the optimal settings for PFA (delivery, design, and dose)? Do these settings differ between normal and highly scarred atria?
What is the effectiveness of a combined thermal and PFA lesions?
Do any unrecognized safety concerns exist for PFA, given the large footprints of the catheters that are currently most frequently used?
Can wearable devices offer reliable monitoring of AF burden after ablation?
Can artificial intelligence be used to tailor individual therapy?
What is the optimal CA strategy for persistent AF?
What are the optimal timing and ablation modality in patients requiring left atrial appendage occlusion (concurrent or staged procedures)?
How can referral patterns improve the level of access to CA to populations with barriers to receiving care?
The above questions focus on the themes of long-term efficacy and safety of ablation in different groups, effects on quality of life, and effects on cardiovascular outcomes (all-cause mortality, CV death, HF hospitalization, and stroke). Subsequent studies are recommended to focus on answering these questions.
Conclusions
Emerging data suggest that first-line ablation therapy for AF is more effective than AAR in maintaining SR, with minimal complication rates. Early rhythm control (<12 months after diagnosis) in selected patients improves clinical outcomes, including mortality, stroke, or heart failure hospitalization. Advanced technologies and techniques will continue to improve ablation success and decrease complications. A multidisciplinary approach is needed, with risk prevention, life-style modification, and early treatment of AF as the first-line therapy in selected patients.