Introduction
Cardiac resynchronization therapy (CRT) has been shown to improve left ventricular (LV) function, reduce heart failure hospitalizations, and reduce mortality in selected heart failure (HF) patients with nonischemic cardiomyopathy (NICM) with left bundle branch block (LBBB) [1–4]. However, a major issue with CRT is that when patients are selected according to current guidelines, about 20–40% still do not benefit [5, 6]. Traditional predictors of CRT response, including echocardiographic assessment of dyssynchrony, have proved disappointing in multicenter studies [7].
Insights are needed into the pathogenesis of HF to accurately select CRT candidates [8]. Previous studies suggested that LBBB is a reversible cause of NICM with HF. In a prospective study involving 29 patients with dilated cardiomyopathy and LBBB [9], LV dysfunction was completely reversed after CRT in a small but important subset (17%) of the patients, first giving rise to the new concept of LBBB-induced cardiomyopathy. Vaillant et al. [10] reported that HF may develop over a mean of 11.6 years of LBBB, and LBBB-induced cardiomyopathy was identified in 1.6% of 375 patients who received CRT. The results showed that improvement of LV structure and function can be achieved by CRT in those patients.
Although the concept of LBBB-induced cardiomyopathy was proposed, it is difficult or impossible to determine definitively the causal relation between cardiomyopathy and LBBB only by clinical records. Consecutive clinical documents in many years are needed, especially in the asymptomatic period, which cannot be saved well by most HF patients. The association between HF pathogenesis and CRT response should be explained integrally, and practical criteria for selecting CRT candidates are needed.
Here our proposal is that the temporal relation between cardiomyopathy and LBBB could be a key factor for CRT response, and the chronological order can be identified by different contraction patterns in cardiovascular magnetic resonance (CMR), which could be a principal consideration for selecting candidates for CRT. We present two patients as evidence: one had LBBB-induced cardiomyopathy with specific features in CMR and had good response to CRT; the other had cardiomyopathy-induced LBBB with different CMR characteristics and poor response to CRT.
Methods
All patients in Beijing Anzhen Hospital with HF and for whom CMR images were available were reviewed. Cardiomyopathy and LBBB were diagnosed in 117 patients, 24 of whom received CRT. There were two patients who had a clear temporal relation between cardiomyopathy and LBBB. Ethics approval was obtained from the Human Research Ethics Committees of Beijing Anzhen Hospital, and informed consent was sought from individual patients for their agreement to participate in long-term follow-up.
Inclusion Criteria and Data Acquisition
LBBB was defined as follows: QRS duration 120 ms or greater, QS or rS in lead V1, broad (frequently notched or slurred) R waves in leads V5 and V6, absent Q waves in leads V5 and V6 [11].
NICM with HF was diagnosed by echocardiography according to the following criteria: LV ejection fraction (LVEF) of 40% or less, LV end-diastolic diameter (LVEDD) of 55 mm or greater, New York Heart Association (NYHA) functional class III or IV, no evidence of features or history suggesting coronary artery disease or a potential reversible cause of NICM, including alcoholic cardiomyopathy, thyrocardiomyopathy, myocarditis and tachycardiomyopathy were excluded.
The patients’ baseline characteristics, including age, sex, and history of coronary artery disease, hypertension, and diabetes, were collected. The onsets of LBBB, cardiomyopathy, and HF were retrospectively ascertained by review of medical records including ECG and echocardiography recordings.
CMR Analysis
All CMR scans were performed before CRT at admission. The patients were scanned with a 3.0-T magnetic resonance scanner (Verio, Siemens Healthcare, Germany). All analyses were performed with CVI42 (version 5.3, Circle Cardiovascular Imaging). A customized software program was used for semiautomated analysis of LV strain. LV endocardial and epicardial borders were manually traced in end-diastolic short-axis cine views and contractile strain plots for each LV segment were automatically generated.
For CMR modalities, the left ventricle was divided into 17 segments according to the American Heart Association LV segmentation model. LV wall thickness at the end of systole and the end of diastole, LV septal-to-lateral thickness ratio, and LV wall thickening were obtained at the midventricular level. The presence of abnormal late gadolinium enhancement in the LV myocardium was visually detected and was also quantitated by CVI42. In addition, the circumferential strain, representing myocardial shortening/stretching in the short-axis plane, was assessed. Regional stretching is expressed as a positive value and shortening is expressed as a negative value. From these data, peak strain, time to peak strain, strain rate, and peak shortening delay (the delay of the first peak circumferential strain between the septal wall segment and the lateral wall segment) were calculated [12].
In-Hospital Management
The patients continued to have NYHA functional class III or IV HF with LVEF less than 40% despite optimal medical treatment, so CRT was recommended for these symptomatic HF patients in sinus rhythm with a QRS duration of 150 ms or greater and LBBB (class I, level A) [6]. After consent had been obtained, CRT devices were implanted transvenously. The LV lead was implanted in the lateral or the posterolateral region of the left ventricle, ideally in the midventricular region rather than the basal or apical region. The implantation of a bipolar LV lead was recommended to enable all four LV pacing configurations so as to avoid phrenic nerve stimulation and/or to maximize the LV pacing threshold in the final lead position. Systematic efforts were made to obtain the narrowest biventricular paced QRS complex during the implantation procedure and initial programming.
Follow-up and Outcome Assessment
All patients received optimal medical treatment after CRT and were followed up by face-to-face interviews in outpatient clinics. At each follow-up visit, echocardiography was used to examine the changes in LVEDD and LVEF. The patients’ symptoms were also evaluated and were graded according to NYHA functional class. CRT response criteria were defined as at least a 15% reduction in LVEDD, a 5% absolute increase in LVEF, and change to at least one NYHA class lower on the basis of prior studies that assessed response to CRT [13, 14].
Results
Baseline Characteristics
The baseline characteristics of the patients are detailed in Table 1. In patient 1, LBBB had been diagnosed 23 years before, at which time echocardiography showed her LVEDD was 49 mm and her LVEF was 61%; cardiomyopathy with HF appeared 9 years later (seemingly induced by LBBB). In patient 2, cardiomyopathy and HF had been diagnosed 8 years before. His LVEF was 40% and his LVEDD was 70 mm. The ECG then showed a normal QRS duration of 100 ms, and LBBB was present at the latest admission. ECGs at different stages are shown in Figure 1.
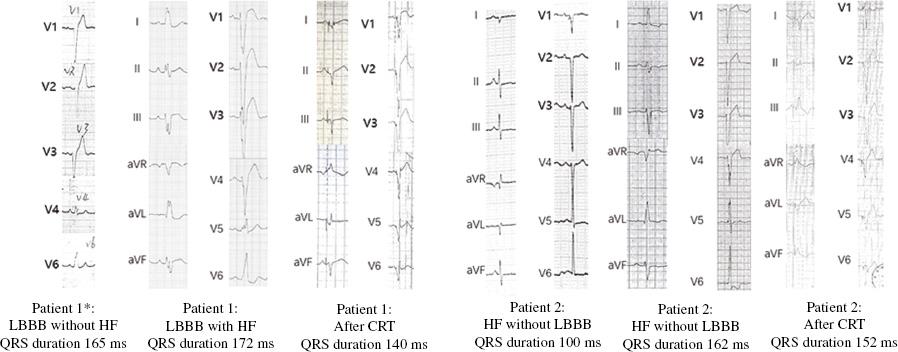
ECGs of the Two Patients Before and After Diagnosis of Cardiomyopathy and Heart Failure.
The asterisk indicates that the limb leads ECG of patient 1 was missing (23 years before). CRT, cardiac resynchronization therapy; HF, heart failure; LBBB, left bundle branch block.
Main Clinical and Echocardiographic Characteristics of Individual Patients at the Baseline.
Patient 1 | Patient 2 | |
---|---|---|
Sex | Female | Male |
Age (years) | 57 | 57 |
CAD | No | No |
Hypertension | No | No |
Diabetes | No | No |
QRS duration (ms) | 172 | 162 |
LVEF (%) | 23 | 29 |
LVEDD (mm) | 77 | 85 |
Time of LBBB diagnosis | 23 years before | At admission |
Time of cardiomyopathy and HF diagnosis | 14 years before | 8 years before |
Time between diagnosis of LBBB and cardiomyopathy (years) | 9 | −8 |
Time between diagnosis of HF and CRT device implantation (years) | 14 | 8 |
CAD, coronary artery disease; CRT, cardiac resynchronization therapy; HF, heart failure; LVEDD, LV end-diastolic diameter, LVEF, left ventricular ejection fraction.
CMR Measurements
CMR measurements of the two patients before CRT device implantation are shown in Table 2 and Figure 2. The LV wall thickness at the end of systole and the wall thickening rate were higher in patient 1, and the ratio of septal-to-lateral thickness was much lower. In addition, the peak circumferential strain for patient 1 was comparatively higher in the lateral and septal walls, and the peak circumferential strain delay between the lateral wall and the septal wall was longer for patient 1. Distinctively different LV deformation patterns in the two patients were seen from CMR tissue tracking (Figure 3).
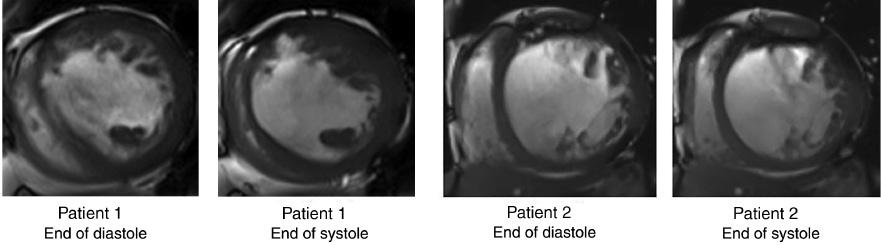
Cardiovascular Magnetic Resonance Images of the Two Patients at the End of Systole and the End of Diastole.
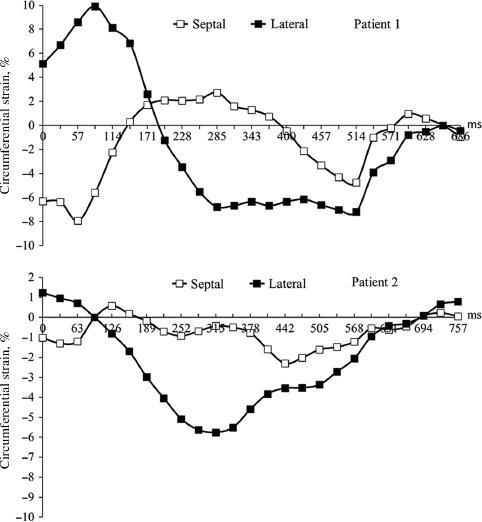
The Instantaneous Strain Curves of Individual Patients from Cardiovascular Magnetic Resonance Tissue Tracking Patient 1 Showed a Specific Contractile Pattern with an Initial Presystolic Septal Contraction (Negative Circumferential Strain) Followed by Stretching and Dyskinesis (Positive Circumferential Strain) of the Septum during the Entire Systole.
For patient 2, the cardiovascular magnetic resonance strain curve showed a significant decreased amplitude of contraction of both the septal segment and the lateral segment.
Cardiovascular Magnetic Resonance Measurements of Individual Patients Before Cardiac Resynchronization Therapy Device Implantation.
Patient 1 | Patient 2 | |||
---|---|---|---|---|
Lateral wall | Septal wall | Lateral wall | Septal wall | |
Wall thickness at end of diastole (mm) | 7.43 | 5.83 | 5.27 | 9.88 |
Septal-to-lateral thickness ratio | 0.78 | 1.87 | ||
Wall thickness at end of systole (mm) | 9.51 | 5.75 | 5.03 | 9.86 |
Septal-to-lateral thickness ratio | 0.60 | 1.96 | ||
Wall thickening (%) | 30.26 | 0.37 | −1.17 | −0.12 |
Late gadolinium enhancement (%) | 0.8 | 0 | ||
Peak circumferential strain (%)* | −7.26 | −7.95 | −5.75 | −2.31 |
Peak circumferential strain rate (1/s) | −1.48 | 1.16 | −0.40 | 0.38 |
Time to peak circumferential strain (ms) | 515.74 | 57.30 | 316.69 | 443.36 |
Peak circumferential strain delay† (ms) | 458.44 | −126.67 |
*A negative value of circumferential strain indicates shortening of the regional wall.
†Peak circumferential strain delay indicates the time between the peak circumferential strain of the lateral wall and the peak circumferential strain of the septal wall.
Outcomes
During follow-up, the echocardiographic and NYHA classes of the two patients were assessed. Patient 1 demonstrated a good response to CRT; in contrast, patient 2 had no response to CRT (Table 3).
Echocardiographic and Clinical Parameters for Cardiac Resynchronization Therapy (CRT) Response for the Two Patients at each Follow-Up Visit.
Patient 1 | Patient 2 | |||||||
---|---|---|---|---|---|---|---|---|
Before CRT* | 6 months | 12 months | 18 months | Before CRT* | 6 months | 12 months | 24 months | |
LVEF (%) | 23 | 30 | 29 | 32 | 29 | 29 | 26 | 22 |
LVEDD (mm) | 77 | 66 | 62 | 63 | 85 | 88 | 85 | 84 |
NYHA class | III | II | II | II | III | III | III | III |
LVEDD, left ventricular end diastolic dimension; LVEF, left ventricular ejection fraction; NYHA New York Heart Association.
*The baseline time was within 1 week before CRT device implantation at admission.
Discussion
The cases we report here support the concept that patients with LBBB-induced cardiomyopathy will have a positive response to CRT, while patients with cardiomyopathy-induced LBBB will have a poorer response to CRT. Moreover, distinctively different cardiac contractility and LV deformation patterns can be recognized by CMR tissue tracking in those two patients. CMR findings are helpful to elucidate the underlying temporal relation between LBBB and cardiomyopathy, to select candidates for CRT, and to predict the prognosis.
It has been suggested that LBBB induces myocardial remodeling that includes asymmetric hypertrophy, fiber disarray, increased myocardial catecholamine concentrations, and altered perfusion distribution, thus contributing to LV dilatation and dysfunction [15]. Meanwhile, increased wall stress, a longer conduction pathway, molecular/cellular changes, and fibrosis in HF patients may impair the conductive tissue, which conversely aggravate asynchronous electrical activation [16]. As a result, the conduction disturbance and the cardiac remodeling seen in HF patients with NICM and LBBB interact to produce a vicious cycle [17].
Mechanisms of LBBB-Induced Cardiomyopathy
With LBBB, the LV motion shows significant delay and is uncoordinated, with paradoxical septal motion, LV remodeling, and hemodynamic deterioration [18]. The anomalous contraction induces redistribution of cardiac mass, the early-activated regions becoming significantly thinner because of the low wall stress than the late activated regions, which are contracting against a higher wall stress, which results in the disuse atrophy of the LV septum and relative hypertrophy of the lateral wall [19] and leads to chronic LV dilatation (Figure 4). If the LV activation sequence is corrected by CRT, the mechanical dyssynchrony and cardiac remodeling can be reversed and patients will have a good or even “super” response to CRT (Figure 5).
Mechanisms of Dilated Cardiomyopathy–Induced LBBB
However, in patients with preexisting cardiomyopathy, HF develops first and subsequently evolves with conduction disturbances. It is assumed that the underlying disease process, which induces not only LV dilation and LVEF reduction, may also be associated with myocardial fibrosis, and cellular uncoupling is likely to produce LBBB [17, 20]. In patients with cardiomyopathy-induced LBBB, the LV dysfunction is due to myocardial degeneration and fibrosis rather than asynchronous electrical activation (Figure 4). Even though the activation sequence can be corrected by CRT, there is diffuse akinesia in the left ventricle, and the LV function cannot be restored; therefore, it is likely such patients will not respond to CRT. Besides, as all the cardiomyocytes, including the conduction system, are affected by degeneration, the ECG in those patients may not have the typical LBBB morphology.
Assessment of the LV Contraction Patterns in HF Patients with LBBB
To clarify whether LV dysfunction is caused by myocardial akinesia (underlying cardiomyopathy) or is secondary to asynchronous electrical activation (LBBB), CMR circumferential strain was applied to assess the LV contraction pattern [21]. Patient 1 exhibited an initial negative strain in the septal segment reflecting presystolic contraction, followed by a positive strain reflecting stretching and dyskinesis of the septum, while the compliant lateral wall was firstly inappropriately stretched (positive strain) during septal contraction and the peak systolic circumferential strain (negative strain) occurred later (Figure 2). The hemodynamic deterioration appears to be due to the paradoxical systolic septal motion and loss of the interventricular septal contribution to ejection fraction, indicative of a primary electrical abnormality. Therefore, the correction of the ventricular activation sequence by CRT improved both LV function and LV structure in this patient. However, patient 2 showed no dyskinesis but reduced amplitude of septal strain, with attenuated thickness of the myocardial wall and little wall thickening during systole. The results suggest that LV dysfunction was potentially related to electromechanical uncoupling from cardiomyopathy instead of specific conduction system disease, and as expected the patient had no response to CRT.
It has been recognized that LBBB in HF can consist of several different activation patterns [22, 23], which are not apparent on the surface ECG. Using noncontact mapping, Fung et al. [24] found some patients had preserved left bundle activation despite LBBB on ECG, whereas others had either a conduction block during LV activation or homogeneous depolarization propagation within the left ventricle. The latest segment of activation was located in either the lateral or the posterior region. These variables appear to be related to the outcome with CRT.
Several imaging modalities were also advocated to assess the different LV contraction patterns in LBBB patients [25–28]. In a two-dimensional LV longitudinal strain study by echocardiography speckle tracking, a typical LBBB strain pattern showed early contraction in the septal wall and prestretching of the opposing wall, followed by late contraction, which is highly predictive of response to CRT [25]. Likewise, a U-shaped LV contraction pattern in patients with LBBB was identified by CMR cine imaging, which might be explained by there being a relatively thin anterior part of the septum, due to dyssynchrony-induced asymmetric atrophy [19]. The rates of CRT response in those with a U-shaped pattern were significantly greater at 6 months [26–28]. By comparison, the other pattern was characterized by a short transseptal delay and homogeneous slow LV activation toward the lateral wall, which is associated with a poorer response to CRT [26–28]. Nevertheless, the relation between the CMR features and the chronological order of LBBB and cardiomyopathy was not noted.
Study Limitations
Firstly, this is a small series of patients to illustrate the temporal relation between LBBB and cardiomyopathy and response to CRT, which is clearly inadequate to draw a statistically powerful conclusion. Secondly, as an inherent deficiency of the retrospective study design, more detailed clinical documents cannot be provided to determine the causality relation of LBBB and cardiomyopathy. In addition, because CMR is not feasible after CRT device implantation, LV circumferential strain could not be assessed and compared with the preimplantation value. Despite these disadvantages, it can be seen from the literature review that the theory we propose is reasonable and has real importance for selecting CRT candidates. Large-sample prospective studies are needed to address further issues.
Conclusion
The temporal relation between cardiomyopathy and LBBB could be a determinant for CRT response. CMR tissue tracking, which can reveal the temporal relation, may be a useful tool for predicting CRT response. The concept provided a reasonable explanation for the CRT response and has real importance for selecting CRT candidates. However, only two patients with a clear temporal relation between cardiomyopathy and LBBB were identified in this study. Comprehensive records of patients’ medical history and HF progression could be helpful in future studies addressing this issue, Larger prospective clinical trials are needed to study the prevalence of, time course of, and risk factors for LBBB-induced cardiomyopathy.