Introduction
As a powerful noninvasive cardiovascular imaging modality, cardiac magnetic resonance (CMR) imaging plays an important role in the diagnosis and management of cardiovascular diseases. CMR imaging has many advantages in cardiac imaging and is considered a “one-stop shop” to provide comprehensive information on the heart. Recently, dedicated guidelines or consensus on CMR scanning, postprocessing, reporting, and clinical appropriateness [1–4] have been published, providing standardized guidance for the clinical utility of CMR imaging. CMR imaging is emerging in Chinese hospitals and has a promising future. This article will briefly describe the clinical application of CMR imaging in China and discuss obstacles for its future development.
History and Current Status of Clinical CMR Imaging in China
The clinical application of CMR imaging was initiated in the late 1980s [5, 6], when the clinical feasibility of CMR imaging was validated to show cardiac structure. In the late 1990s, the clinical role of CMR imaging became important because of the use of steady-state free precession sequence and LGE. In China, the earliest application of CMR imaging in patients was in Nanfang Hospital, Guangzhou, in the 1980s. Then several reports of CMR studies were published at the end of the 1980s and in the early 1990s [5–7]. However, real-word practice of CMR imaging in the clinical setting in China was very rare, especially in the late 1990s to the early 2000s, when there were very sporadic clinical reports. Clinical CMR imaging was available in only a very limited number of hospitals; for example Fuwai Hospital [8, 9]. However, the situation has begun to change gradually in the past decade; more and more physicians in radiology and cardiology departments have been promoting the use of CMR imaging in clinical practice. On the basis of a brief survey conducted in 2015 to investigate current CMR imaging practice in mainland China, 77 of the 108 participating hospitals reported that they routinely perform clinical CMR imaging. Most of them (63, 82%) were university or academic hospitals. About half of these hospitals performed 50–200 scans per year and only five centers performed more than 500 scans per year. For example, the number of clinical CMR scans in West China Hospital increased from 20 per year to more than 1000 per year from 2009 to 2015, but this still lags far behind clinical demands. The common reasons for CMR imaging are suspected cardiomyopathies, mostly nonischemic, including hypertrophic cardiomyopathy (HCM; 24.6%), dilated cardiomyopathy (DCM; 17.9%), amyloidosis (2.5%), restrictive cardiomyopathy (2.1%), arrhythmogenic right ventricular cardiomyopathy (ARVC; 1.1%), left ventricular noncompaction (0.4%), and Fabry disease (0.4%); only 21.1% of patients were referred for the evaluation of viability after myocardial infarction [10]. The major referral reasons are similar among high-volume centers. In addition, the survey showed that there are state-of-the-art scanners in most hospitals, which provide a good means to promote the clinical utility of CMR imaging.
Clinical Role of CMR Imaging in China
CMR Imaging in Ischemic Heart Disease
Ischemic heart disease is a major cardiovascular disease in China. There are 2 million patients with myocardial infarction and almost 500,000 new cases of myocardial infarction annually [11]. CMR imaging has robust ability in the diagnosis and risk stratification of ischemic heart disease by perfusion, viability, and angiography.
Stress CMR imaging has been used to detect ischemia in coronary artery disease, and has been demonstrated to be better than myocardial perfusion single photon emission computed tomography. However, the extra needs of stress-inducing medication, continuous monitoring devices, and on-site physicians can restrict its routine clinical application in China. From a search for “stress CMR” and “China” in all available databases, including PubMed, Ovid, and Chinese Wanfang databases, there are very few reports on stress CMR imaging performed in patients with suspected ischemic heart disease. Pan et al. [12] performed a clinical study in 71 patients using quantitative stress perfusion CMR imaging and found that myocardial transmural perfusion gradient is a better parameter than conventional transmural perfusion analysis to detect myocardial ischemia when compared with fractional flow reserve.
In 2000 Kim et al. [13] reported a landmark study that showed the transmural extent of infarct assessment by late gadolinium enhancement (LGE) could be a novel parameter for myocardial viability. Potentially, viability evaluation by means of LGE could be used to guide revascularization in ischemic heart disease. In China, Li et al. [14] evaluated the effect of percutaneous coronary intervention on left ventricular function in patients with myocardial infarction assessed by LGE and found results similar to those of Kim et al. Additional studies confirmed that the ability of CMR imaging to identify viable myocardium was not inferior to that of single photon emission computed tomography [15, 16]. The health care cost for cardiovascular diseases has largely increased in recent years, with a total hospitalization expenditure of 40 billion yuan, which is estimated to be 1.64% of the national health expenditures [17]. Although it helps to improve the cost-effectiveness of myocardial revascularization, myocardial viability evaluation by CMR imaging is still underused because to its low availability in China. In addition to LGE, recent studies have revealed that the extracellular volume (ECV) fraction may serve as a promising alternative parameter in assessing regional myocardial injury that could predict recovery after revascularization. Because of its quantitative nature, ECV fraction would be highly reliable and reproducible. Chen et al. [18] reported in a small cohort of patients with chronic total coronary occlusion that the ECV fraction provided a measure additional to delayed enhancement imaging in assessing functional recovery after revascularization. They proposed a novel risk stratification model, including myocardial necrosis and functional abnormalities derived from CMR imaging in ST segment elevation myocardial infarction patients, that could predict the major cardiovascular adverse events at 90-day follow-up [19].
Coronary magnetic resonance angiography (CMRA) was first introduced in 1987 [20]. CMRA shows good accuracy in the diagnosis of coronary stenosis with the development of imaging techniques. It remains a component of the imaging protocol for ischemic heart disease, especially for patients allergic to conventional contrast media. Some small-scale clinical trials in China have demonstrated that contrast-enhanced whole-heart CMRA using a spoiled gradient echo sequence at 3.0 T provided imaging with superior accuracy, relatively high spatial resolution, and a short imaging time. By using this method, Yang et al. [21] demonstrated the feasibility of accurate detection of significant stenosis in coronary arterial segments larger than 1.5 mm. Chen et al. [22] suggested that image quality can be further improved by sublingual nitroglycerin and abdominal banding. One study that combined CMRA with 3D volume-targeted thin-slab fast imaging employing steady-state acquisition (FIESTA) found a similar accuracy in detecting proximal coronary stenosis between CMRA and computed tomograph angiography [23]. Jin et al. [24] compared volume-targeted acquisition with whole-heart acquisition in 1.5-T free-breathing 3D CMRA and found that right coronary artery/left circumflex artery–targeted and left coronary artery/left anterior descending coronary artery–targeted acquisition yielded higher vessel sharpness and overall image quality [24]. The whole-heart method was superior to the volume-targeted method in the visualization of the posterior descending artery. Therefore the different methods of acquiring CMRA images are not competing imaging approaches but have different indications for clinical use. It has been reported that coronary anatomy, myocardial damage, and viability information can be feasibly acquired in one CMR examination in some Chinese heart centers [25].
CMR Imaging in Nonischemic Cardiomyopathy
Cardiomyopathy is a heterogeneous group of diseases. CMR imaging has been established as a robust technique in the evaluation of cardiomyopathies. CMR imaging not only provides comprehensive information on structure and function, but also performs tissue characterization to establish the cause of cardiomyopathy. Not surprisingly, further investigation on nonischemic cardiomyopathies is the most common referral reason for CMR imaging in China.
Dilated Cardiomyopathy
Dilated cardiomyopathy (DCM) is a common cause of heart failure and is characterized by a dilated left ventricle with impaired systolic function in the absence of coronary artery disease and other nonmyocardial causes of dilatation. Left ventricular volumes and left ventricular ejection fraction provide fundamental measures of function and serve as strong prognostic indicators for patients with DCM. CMR imaging is the gold standard for the measurement of ventricular function. More importantly, LGE-CMR imaging, as a gold standard for myocardial scar, is widely accepted in the differential diagnosis of DCM as well as for outcome prediction. In DCM patients, a midline scar, especially in the interventricular septum, is a specific presentation in CMR imaging (Figure 1). In a DCM cohort study, Li et al. [26] repotted that DCM patients with diffuse LGE had higher mortality than patients with LGE in the interventricular septum or LGE in a location other than the interventricular septum. In another prospective study, Lu et al. [27] found that fat deposition is a common phenomenon in DCM, and is associated with fibrosis volume and left ventricular function. However, the cause and prognostic significance need to be further explored. Studies have compared CMR features of isolated left ventricular noncompaction and DCM in Chinese adults and demonstrated some morphological and functional differences; for example, a higher end-diastolic noncompaction/compaction ratio in patients with left ventricular noncompaction [28, 29].
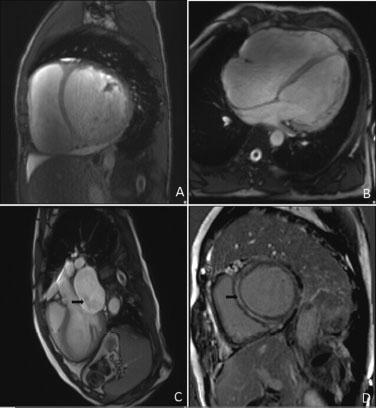
The CMR Images of DCM.
A and B are short-axis and 4 chamber view of cine image showing dilated cardiac chambers, respectively. Additionally, A shows pericardial effusion. C is 3 chamber view of cine image which shows systolic mitral regurgitation (black arrow). D is short-axis of LGE image which shows midline hyperenhancement on LGE in anterior septum, septum and inferior wall (black arrow).
Hypertrophic Cardiomyopathy
Hypertrophic cardiomyopathy (HCM) is a genetic cardiac disease characterized by various degrees of myocardial hypertrophy. The estimated prevalence of HCM in China is 0.13% [30], and therefore at least 1 million patients have this disease. Because of high-quality imaging (Figure 2), CMR imaging was used to investigate HCM patients in China more than 2 decades ago.
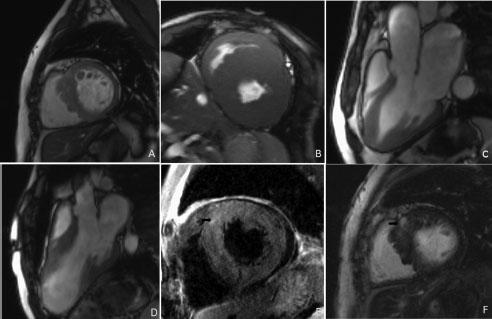
The CMR Images of HCM.
Both A and B are short-axis view of cine images. A demonstrates asymmetric hypertrophy of the septum. B shows homogenous hypertrophy of LV and RV is also involved. Cine images in 3 chambers view (C and D) show some special phenotypes of HCM including apical hypertrophy and mid left ventricular hypertrophy with thinning myocardium and aneurysm at the apical segments. T2-weighted image in short axis view (E) reveals high signal area which indicates myocardial edema in the anterior insertions of the RV (black arrow). LGE short-axis view (F) the anterior insertions of the RV (black arrow).
CMR imaging can clearly depict the morphology of the septum and left ventricular outflow tract (LVOT). The hypertrophic patterns of the septum are critical determinants of blood flow and function, but are variable. Zhong et al. [31] developed an independent coordinate method to quantify the regional shape of the left ventricle in terms of curvature by CMR imaging to identify the morphology subtype of HCM. Furthermore, Yan et al. [32] focused on the long-term outcome of HCM patients with mid-ventricular obstruction as a rare form of HCM. The results indicated that in Chinese patients, mid-ventricular obstructive HCM is associated with an unfavorable prognosis in terms of cardiovascular death. Additional studies investigated ventricular function in Chinese HCM patients by means of novel parameters and found that longitudinal strain was commonly impaired [33] and that right ventricular systolic function was associated with symptomatic severity [34].
Myocardial fibrosis is a very important pathologic characteristic of HCM, and can be observed with LGE-CMR imaging. LGE at the anterior and inferior insertions of the right ventricle is the most typical replacement fibrosis pattern. Apical HCM (ApHCM) is a special subtype of HCM for which there are more patients in the Far East. Guo et al. [35] observed a special pattern of LGE distribution in Chinese ApHCM patients. LGE was found not only in the thickened apex but also in other left ventricular segments irrespective of the presence or absence of hypertrophy. Studies have demonstrated that LGE is an independent risk factor for sudden death, heart failure progression, and ventricular arrhythmia in HCM patients, which was also revealed in Chinese patients [36]. Nevertheless, this relationship has not been confirmed in Chinese ApHCM patients [35].
Percutaneous transluminal septal myocardial ablation (PTSMA) as an alternative to surgical myectomy to relieve LVOT obstruction was first reported in 1995 [37]. Thousands of HCM patients have received this treatment in China. Lu et al. [38] reported good correlations between the reduction of postoperative LVOT gradient and the thickness of the basal anterior segment, the thickness of the basal anteroseptal segment, and the total thickness of these two segments. Moreover, they investigated and supported the value of the use of CMR imaging during patient selection for the PTSMA procedure. Yuan et al. [39] demonstrated the feasibility of CMR imaging in the short-term and long-term follow-up after PTSMA. Another 16-month follow-up study involving CMR imaging revealed that PTSMA not only significantly reduced LVOT obstruction but also reversed biventricular remodeling in terms of decreased right ventricular and left ventricular masses and increased right ventricular and left ventricular end-diastolic volumes [40]. In addition, significant and sustained improvement in left ventricular diastolic function has been observed after PTSMA [41].
Arrhythmogenic Right Ventricular Cardiomyopathy
Arrhythmogenic right ventricular cardiomyopathy (ARVC) is an inherited disease that primarily affects the right ventricle. The clinical presentation is usually malignant arrhythmia or sudden cardiac death. A 2010 ARVC task force defined right ventricular kinesis and right ventricular ejection fraction measured by CMR imaging as major criteria for the diagnosis of ARVC [42], in which CMR imaging was strongly recommended as the optimal imaging modality to significantly increase the sensitivity of detection (Figure 3). However, only two small cohort studies have validated the feasibility of CMR imaging for the diagnosis of ARVC in Chinese patients [43–45]. The prominent clinical profile of Chinese patients with ARVC includes relatively low incidence of premature sudden death and uncommon left ventricular involvement [43]. Cheng et al. [46] reported that N-terminal prohormone of brain natriuretic peptide was associated with the extent of right ventricular dilatation and dysfunction measured by CMR imaging in patients with ARVC, and may be considered a prognostic marker. In addition, Ma et al. [47] found that right ventricular outflow tract area, right ventricular end-diastolic volume, and end-systolic volume measured by CMR imaging were positively correlated to the extent of QRS dispersion on a Holter recording. In a cohort study that included 193 Chinese patients with ARVC, 10 intracardiac thrombi were identified in eight patients; most of them (7 of 10) were found in the right ventricular apex [48]. Multivariate analysis showed that female sex and left ventricular dysfunction are independently associated with a high risk of ventricular thrombosis in ARVC.
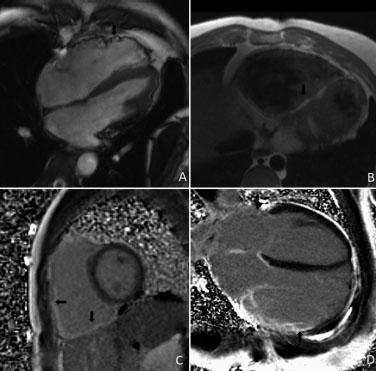
The CMR Images of ARVC.
Four-chamber cine image (A) shows marked enlargement of the RV, impairment of global RV function, segmental minimal aneurysm of the RV wall (black arrow). Short-axial T1-weighted fast spin-echo image (B) detects fatty infiltration of RV (black arrow). Both C and D are LGE images with PSIR which demonstrate the hyperenhancement of RV (black arrow).
CMR Imaging in Myocarditis
Myocarditis is an inflammatory myocardial disease that may be caused by different pathogens and triggers. The diagnosis of myocarditis remains a difficult clinical scenario, since the clinical spectrum of myocarditis extends from mild or no symptoms to acute cardiogenic shock. CMR imaging is currently the best noninvasive imaging modality to confirm the diagnosis of myocarditis, with its ability to detect focal inflammation and scarring (Figure 4). In the United States and other Western countries, CMR imaging is commonly performed in patients presenting with acute chest pain and raised levels of myocardial injury biomarkers after normal coronary arteries are demonstrated. However, CMR imaging is not yet a routine imaging modality in patients suspected of having myocarditis, probably because of the long waiting time and the high cost of the examination. In a cohort study that enrolled only 30 patients with suspected myocarditis, the diagnostic values of different CMR scan methods (edema imaging, global relative enhancement, LGE) involved in the Lake Louise criteria [49] were compared. The results reconfirmed that CMR imaging is an excellent imaging modality. Liu et al. [50] described the variety of CMR findings in children with myocarditis, including regional or global myocardial signal increase in T2-weighted images and early gadolinium enhancement and LGE in T1-weighted images. In addition, some complicated cases of myocarditis detected by CMR imaging were reported [51, 52].
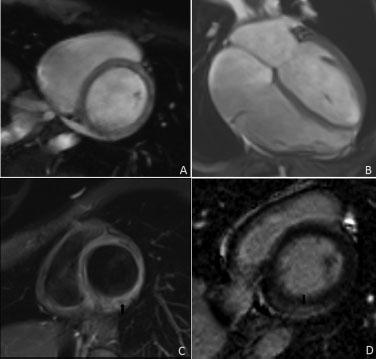
The CMR Images of Myocarditis.
Both the short-axis (A) and the four-chamber (B) cine image show the mildly enlargement of cardiac chambers. Moreover, pericardial effusion can also be detected in myocarditis (B). T2-weighted image (C) show high signal which indicate myocardial edema in inferolateral region (black arrow). LGE image (D) show subepicardial layer of hyperenhancement in inferior wall of LV (black arrow).
CMR Imaging in Congenital Heart Disease
CMR imaging is an ideal test for the initial diagnosis and follow-up of congenital heart disease because it is noninvasive and does not expose patients to ionizing radiation. Recently, we demonstrated by using CMR imaging in a group of patients with Ebstein anomaly that cone reconstruction as a novel surgical procedure for tricuspid valve repair yielded a good short-term survival [53]. Cone reconstruction reduced functional right ventricular volume, improved right ventricular global synchronization, and restored right ventricular geometry. Contrast-enhanced magnetic resonance angiography (MRA) is a noninvasive modality for unlimited visualization of the aortic arch; however, only a few studies have demonstrated the feasibility of this imaging modality for the detection of congenital aortic arch anomalies in Chinese patients [54, 55]. Safety issues related to gadolinium-based contrast agents, in particular those with reduced renal function, are major concerns. Therefore Chang et al. [56] adopted a novel unenhanced CMR technique based on 3D steady-state free precession to compare it with contrast-enhanced MRA. This novel technique provides powerful complementary information for patients who undergo contrast-enhanced MRA, especially at the ventriculoarterial connection site.
New CMR Techniques for Clinical Research in China
In recent years, quantitative CMR techniques, such as T1, T2, and T2* mapping, have shown their potential unique clinical and research values. Gao et al. [57] reported that the native myocardial T1 values were significantly increased in hypothyroidism patients compared with healthy controls and that increased T1 values were correlated with free triiodothyronine concentration and cardiac function impairment. The study indicated that diffuse myocardial fibrosis was common in hypothyroidism patients. Myocardial ECV fraction is defined as the percentage of extracellular space, which is measured by use of myocardial T1 imaging before and after contrast agent administration, and calibrated with hematocrit. Chen et al. [18] recently demonstrated that ECV fraction was able to assess myocardial injury and angiographic collateral flow in patients with chronic total coronary occlusion. In addition, ECV fraction could predict functional recovery after revascularization in these patients [18]. The T2 value reflects the content of water in the myocardium, which is considered a sensitive parameter to detect myocardial edema, a common pathophysiologic feature in some inflammatory myocardial diseases or acute myocardial injury. T2 mapping techniques as a quantitative technique can provide a reliable, discrete, and quantifiable myocardial T2 value. An et al. [58] reported that the T2 value was significantly higher in the myocardial edema region than in distal normal myocardium in patients with myocardial infarction. That study confirmed the value of quantitative T2 mapping in identifying the myocardial edema region in Chinese patients with acute myocardial infarction [58]. T2* mapping is a sensitive quantitative technique for detecting myocardial iron deposition (i.e., hemochromatosis), which is not uncommon in some regions of southern China. Although T2* mapping has been recommended to monitor myocardial involvement in patients with thalassemia or chronic anemia who received multiple blood transfusions, T2* mapping in the heart is not underscored in China.
The Challenges and Future Direction of Clinical CMR Imaging in China
Firstly, our recent survey demonstrated that very few hospitals have the ability to perform clinical CMR imaging programs, although advanced MRI scanners are available in a lot more hospitals. The number of hospitals with a CMR examination volume of more than 200 cases per year is low. Secondly, few cardiologists have been trained in CMR imaging, whereas most of the scanning and reporting work is done by technicians and radiologists, who are less experienced in interpreting cardiac anatomy and hemodynamics. Lastly, there was no evidence of guidelines or consensus for the clinical use of CMR imaging in China until the first Chinese expert consensus on CMR application in cardiomyopathy was released in 2015 [59]. This consensus defined the possible clinical roles of CMR imaging and potential indications for application of CMR imaging, and provides useful instructions for referring patients for CMR imaging and helps clinicians to understand the unique role of CMR imaging in cardiomyopathy. With regard to future developments, standardizing the CMR protocols should be the first step, including scanning, postprocessing, and reporting, to ensure a high quality of clinical CMR imaging. This must be followed by the establishment of a multidisciplinary team to enroll more cardiologists who are interested in CMR imaging. Thirdly, more high-quality CMR studies in the Chinese population should be encouraged, in particular in those diseases that are more prevalent in China with different phenotypes within the population.