INTRODUCTION
Inventors of high-brightness blue light-emitting diode (LED) were awarded the 2014 Nobel prize for physics [1]. Silicon carbide (SiC) single crystals, along with sapphire, GaN, and silicon, are one of the most important substrates for high-brightness LED fabrications. Among all processes leading to final LED devices such as crystal growth, slicing, polishing, deposition of functional layers, and packaging, chemical mechanical polishing (CMP) is one of the key steps enabling successful deposition of functional III-V group layers such as GaN (as well as buffer layers).
With the CMP process, pre-polished SiC wafers, with surface roughness at nanometers scale, are subjected to simultaneous chemical and mechanical action in a CMP machine where a loaded substrate is rotated against a polishing pad with slurry continuously feed containing polishing colloids and various chemicals [2–4]. The final surface roughness after CMP is usually less than 0.5 nm, thus enabling an atomic-scale smoothness to be achieved [5]. However, owing to the extremely high hardness (Mohs’ scale of 9.5) and chemical inertness, the polishing rate of SiC with conventional CMP methods [6,7] using slurry containing silica alumina or ceria colloids under harsh alkaline conditions is low [8–11]. At the same time, surface scratches are inevitable owing to a rather high hardness of polishing colloid particles.
Recently, oxidative solutions such as hydrogen peroxide have been introduced into SiC CMP slurry to explore the possibility of accelerated oxidation and removal, taking advantage of highly reactive OH hydroxyl free radicals released from hydrogen peroxide molecules [8,13,14]. Nevertheless, regardless of progress, the problems of persistent scratches and treating/recycling large amounts of used harmful wastes have yet to be solved. A new method for green, scratch-free CMP processing of SiC substrates is thus highly desired.
Discovery of artemisinin (Qinghaosu) as a revolutionary anti-malarial drug fetched Youyou Tu the 2015 Nobel prize for physiology or medicine [15]. Artemisinin, with a molecular formula of C15H22O5 and with a molecular weight of 282 Da, belongs to sesquiterpene lactone endoperoxide. Researchers generally believe that it is the unique endoperoxide bridges that make artemisinin very effective in curing malaria through iron-mediated release of ·OH free radicals after cleaving endoperoxide bridges [16]. However, unfortunately this special Fenton-like reaction has not been paid due attention outside the pharmaceutical area.
In this study, two seemingly alien areas – artemisinin and polishing – were combined. Soft artemisinin (Qinghaosu) crystals were surprisingly found to effectively polish SiC single crystals even in water as revealed by proof-of-concept scratching experiments using atomic force microscopy (AFM)-based colloidal probe nanotribology strategy [17,18]. The preliminary results reported here will have important implications for developing alternative green and scratch-free polishing methods for hard-brittle substrates.
EXPERIMENTAL
Artemisinin (Qinghaosu) crystals, SiC wafers
Epitaxial (EPI) grade polished single-crystal c-plane 6H-SiC single crystal substrates were 10 mm × 10 mm × 0.2 mm in size (Hefei Ke Jing Materials Technology Co., China). Commercial artemisinin (Qinghaosu) crystals (C15H22O5, CAS No. 63968-64-9) were produced from the extract of leaf Artemisia annua L with the batch number of SWX2015082504 (Xi’an Zebang Biological Technology Co., China). The purity specified by the manufacturer is 99.2% as examined by the high performance liquid chromatography (HPLC) method. The crystals, white needle-like, 20–100 μm in width and 50–500 μm in length as examined with an optical microscopy, were used as-received without further purification.
Wet cleaning of SiC wafers
SiC wafers were first immersed and sonicated in the diluted detergent solution (15PF-x, Deconex Co., Germany) for 40 min, rinsed with copious de-ionized (DI) water (resistivity of 18.2 M/cm), then soaked in the piranha solution (H2SO4 + H2O2 3:1 mixture) for 30 min at 100°C (Caution: This Solution is Dangerous. Handle with Care!), finally rinsed with copious DI water and blown dry with a stream of high-speed nitrogen (≥99.99%) using a nitrogen gun (KITZ, Japan). Notably, the SiC substrates were easier to be critically cleaned than sapphire substrates [19,20]. To avoid silica contamination, polyfluortetraethylene (PTFE) plastic wares were used for each wet cleaning step. Cleaned SiC substrates were stored in a sealed PTFE container prior to tests.
AFM characterization
A MultiMode 8 AFM system (Bruker Co., USA) including a NanoScope V controller was used in contact mode for scratching tests in water, and in the tapping/ScanAsys mode for topographical characterization. The scanner (model AS-130VLR) is capable of 125 μm × 125 μm × 5 μm scan range. All topographical images were captured and saved in the raw format and processed with the free WSXM software [21]. Silicon probes (NSG10, NT-MDT Co., Russia) with a nominal spring constant of 11.5 N/m were used for AFM topographical characterization.
Preparation of artemisinin-modified AFM probe
Tipless silicon probes (All-In-One-TL, Budget Sensors, Bulgaria) with nominal spring constant of 40 N/m were used for preparation of artemisinin modified probe and AFM scratching tests. Artemisinin-modified probes were prepared by gluing, with UV-curable glue (Norland Products Inc., USA), a small artemisinin crystal (or a broken part of larger crystals) of tens of micrometers in size to the cantilever of the tipless probe following the cantilever-moving procedure [22]. Curing of glue was carried out in a home-built UV light unit containing a UV light lamp (UVGL-55, UVP Inc, USA) fixed in a stainless steel container with a cover lid. At the same time, UV light irradiation also cleaned the probe by removing organic contaminants.
AFM scratching test using artemisinin-modified AFM probe in water
The procedure for a typical AFM scratching test is as follows. First, the artemisinin probe was examined by a microscope to ensure the artemisinin crystals were successfully attached to the cantilever. Then the probe was engaged with the substrate in water (with a quartz liquid cell) to scratch at a constant loading force (10.3 μN, the maximum force executed by the cantilever used) over a 5 μm × 5 μm area at 1 Hz for 60 min without break. After disengagement, the substrate was taken out and cleaned with the same cleaning procedure above, and the scratched area was subjected to AFM imaging for topographical analysis. The pH of water was adjusted using NaOH and H2SO4. Analytical grade chemical reagents were used. The temperature of the AFM laboratory was around 25°C and relative humidity was around 20%.
RESULTS AND DISCUSSION
Artemisinin crystals, artemisinin-modified AFM probe
Optical micrograph of a few artemisinin crystals spread on glass slides, as shown in Figure 1a, shows varying size of artemisinin crystals with width from 50 to 100 μm. The crystal glued to the AFM cantilever was generally smaller than 50 μm, i.e., either a crystal smaller than those shown in Figure 1a or a broken part of a larger crystals was used. The artemisinin probe was examined by a microscope before AFM test, Figure 1b shows the side view of a tipless AFM cantilever with an irregular shaped artemisinin crystal successfully glued close to the triangular end of the cantilever. This type of artemisinin crystal-modified AFM probe was used for AFM scratching test in water with a liquid cell. Note that pharmaceutical researchers used the similar drug particle-modified AFM probes, the so-called colloidal drug probes by some, for adhesion studies [23–25].
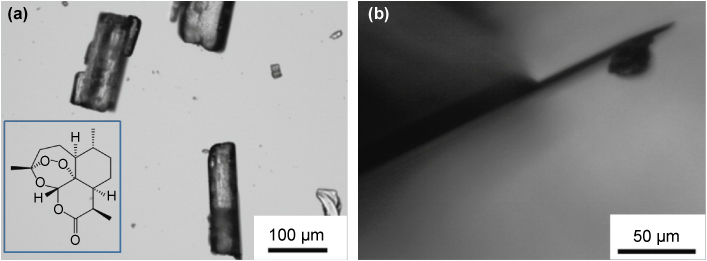
AFM characterization of scratched substrates
AFM topographical image of SiC substrates after being scratched with the artemisinin-modified probe for 60 min in DI water was recorded after the substrate was disengaged with the probe and cleaned. As shown in Figure 2a, even the scratching area was set to as small as 5 μm × 5 μm for this test, multiple squares (four of them) could be found. This was attributed to either simultaneous multiple contacts between irregularly shaped artemisinin crystal and the substrate, or to varying contact asperities caused by gradual wearing of the probe against the substrate during scratching as observed for silica spheres [17]. Notably, besides these scratched areas, a straight scratch line running from the bottom to the top of the image as marked by two white arrows, about 1 μm in width, disappeared within the scratched area. Disappearance of this scratch line which existed in the substrate before the test strongly indicates a removal of substrate materials, and this is clear from the three-dimensionally rendered topographical image (Figure 2b). Line profiles along two lines, right to each other across the scratched area, indicating the maximum depth was ~0.5 nm for the deepest area, whereas some parts were shallower.
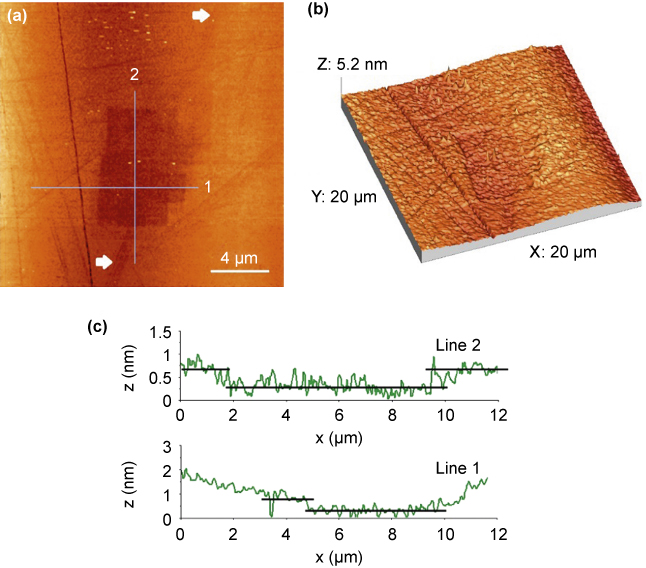
Comparing hardness of artemisinin with SiC and other common abrasives
It is surprising, from traditional CMP process and materials point of view, that very hard SiC with Mohs’ scale of 9.5 can be scratched by a much softer organic molecular crystal such as artemisinin. The quantitative values of hardness of artemisinin are not available both experimentally and theoretically at the moment to the best of our knowledge. We can have the order of magnitude estimate of the relative hardness of SiC, quartz, and artemisinin by comparing their melting point which is closely related to the strength of cohesive bonds: SiC (2,730°C, a covalent crystal), quartz (1,750°C, a covalent and ionic crystal), and artemisinin (152°C, a van der Waals crystal). We can thus safely expect very low hardness of artemisinin crystals – much lower than all common polishing solids such as silica, alumina, a ceria. The hardness of artemisinin may be comparable with other pharmaceutical solids such as ibuprofen [26]. The reasoning about very low hardness of artemisinin thus leads us naturally to conclude that it is impossible to explain the scratching results from a pure mechanical point of view.
Mechanism discussion: decomposition of SiC mediated by mechanically activated ·OH free radicals in water
Therefore, we need to turn to the intrinsic chemical nature of artemisinin to interpret the observed results. We noticed the fact that it is the unique endoperoxide bridge in a artemisinin molecule that makes artemisinin very effective in curing malaria by iron-mediated release of ·OH free radicals through cleaving endoperoxide bridges [16]. However, this special Fenton-like reaction has not been paid due attention outside pharmaceutical areas. It thus occurs to us that the underlying polishing mechanism can be reasonably attributed to activated oxidation and the subsequent removal of SiC substrate materials mediated by the release of highly reactive ·OH free radicals from the endoperoxide bonds under mechanical activation, following the proposed reactions (1) and (2), as illustrated during the AFM scratching test (Figure 3a and b):
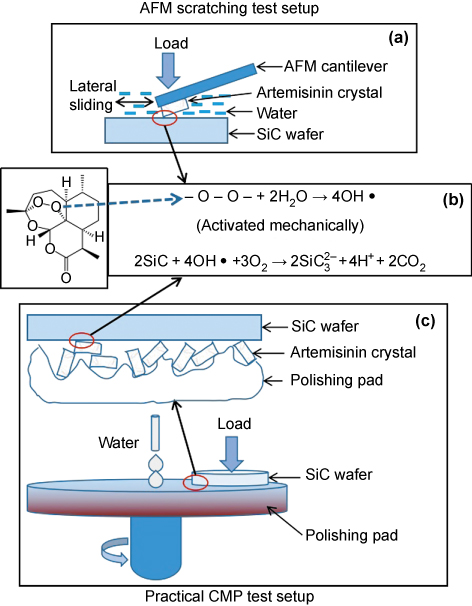
The proposed reaction (2) is not involved with the generation of SiO2, as commonly proposed by others in H2O2-mediated SiC CMP [13,14] where silica particles are capable of mechanically removing SiO2 because artemisinin crystal is too soft to allow a mechanical removal of harder SiO2. Therefore, the proposed reactions favor the removal of SiC through direct decomposition of SiC into soluble species SiO32− instead of solid SiO2. Although the reaction (2) tells that higher pH will facilitate the removal of SiC, our preliminary experiments carried out in different pH conditions (results not shown here) show that acidic or neutral conditions, rather than basic conditions, favor the removal of SiC. But it should be kept in mind that the experimental pH dependence must be taken with caution because of significantly varying particle shape and scratching contact geometry among different tests. Here we only speculate that pH dependence, if confirmed by future results, may correlate with reaction (1) or stability of artemisinin. Clearly, the detailed mechanistic pathway, together with practical CMP tests (Figure 3c), needs a careful and systematic study.
Possible strategy for increasing polishing rate
One may argue that a very low removal rate (0.5 nm/h) and low hardness of artemisinin crystals might render it an impractical candidate for practical CMP of hard materials such as SiC. It is true that, as discussed above, low hardness is intrinsic to molecular solids such as artemisinin, thus leading to a larger contact area, reduced local contact pressure, and fracture during scratching. Therefore, the low removal rate is not unexpected. Frankly speaking, we indeed found that artemisinin crystals attached to the AFM probe wore away markedly after the 60-min scratching test, indicating low hardness compared with silica particles for CMP [17]. However, there are ways to bypass this problem, for example, by mixing artemisinin with other polishing materials such as silica or iron to produce composite particles or the so-called hybrid particles [27]. In this way, artemisinin crystals may act as agents for releasing ·OH free radicals and other materials can take the role of mechanical abrasives and/or Fenton reaction-type activators (for the case of iron).
CONCLUSIONS
Artemisinin (Qinghaosu) crystalline particles were successfully used, for the first time to the best of our knowledge, to effectively polish SiC single crystals even in water as revealed by proof-of-concept scratching experiments using AFM. A material removal rate of ~0.5 nm/h was achieved using the irregular-shaped artemisinin particles (tens of micrometers in size) under a load of ~10 μN. The underlying mechanism is attributed to activated oxidation and subsequent removal of substrate materials owing to the release of highly reactive ·OH free radicals from the endoperoxide bonds under mechanical activation. The preliminary results reported here will have important implications for developing novel alternative green and scratch-free CMP methods for hard-brittle substrates including SiC, diamond, and others.